All published articles of this journal are available on ScienceDirect.
A Narrative Review: Exercise Induces Adipose Browning by Altering the Gut Microbiome Profile
Abstract
Obesity is a condition that is highly associated with chronic diseases. The number of obese individuals is increasing on a global scale every year. Energy intake that is imbalanced with energy expenditure causes the accumulation of excess fat. Regular exercise is part of a lifestyle that can reduce the risk of obesity by inducing adipose browning. One of the mechanisms of exercise in inducing browning is by modifying the gut microbiome profile. This profile may promote adipose browning by maintaining the integrity of the intestinal barrier, glucose homeostasis, regulating energy absorption, and appetite-regulating hormones.
1. INTRODUCTION
The main cause of death has shifted from communicable to non-communicable diseases. As the prevention and treatment of communicable diseases increase, deaths from non-communicable diseases also increase [1]. A lack of physical activity contributes to most chronic diseases, such as hypertension, obesity, hyperglycemia, and hyperlipidemia [2].
An unbalanced diet, wherein the intake of calories exceeds energy expenditure, expands the adipose tissue mass due to adipocyte hypertrophy and adipocyte precursor hyperplasia [3, 4]. White fat accumulation is associated with negative health effects, including obesity, insulin resistance, as well as increasing diabetes mellitus. In prolonged conditions, fat accumulation increases the risk factor for chronic diseases, such as cancer and cardiovascular disease [5, 6].
One of the mechanisms that can increase energy expenditure is browning, which changes white fat into brown or beige fat [7-9]. This process is induced by various factors, one of which is exercise [10-13]. Exercise is part of a lifestyle that helps to prevent various chronic conditions [14]. The mechanism by which exercise induces browning is still being explored. Among the most interesting recent studies is one on the role of gut microbiota in improving metabolic health. The gut microbiome is of particular interest, attributable to its role in energy harvesting [15]. Exercise is among the factors that cause physiological changes in the body, one of which is the gastrointestinal system. The gut microbiome, a collection of more than three million microbiota genes in the human gut, is considered to make a strong contribution to the physiological processes of the digestive tract. The gut microbiome can make a genetic contribution to the overall health of the human body [16-18].
Exercise has been shown to affect the gut microbiome profile by reducing the ratio of Firmicutes to Bacteroidetes, fusobacteria, proteobacteria, and increasing microbiota, which are important for body health, including Bacteroidetes, Verrucomicrobia, and Actinobacteria. Recent research has also proven that exercise can improve metabolic health through the role of short-chain fatty acids (SCFAs). SCFAs have various mechanisms of action, one of which is inducing adipose browning. This review discusses the role of exercise in adipose browning by influencing the gut microbiome profile.
2. ADIPOSE TISSUE AND BROWNING
Adipose tissue occupies about 20-28% of the total body mass. This composition varies and is influenced by gender or specific conditions, such as obesity. In obese individuals, adipose tissue can account for 80% of the total body weight [19-21]. The function of adipose tissue varies depending on its type and anatomic location.
Adipose tissue is classified into white fat tissue (WAT) and brown fat tissue (BAT), more commonly known as “white fat” and “brown fat.” Both have different characteristics and functions. White fat functions in energy storage in the form of triglycerides, while brown fat plays a role in fuel oxidation and heat production [22]. White fat is characterized by large white adipocytes containing unilocular lipid droplets. WAT is associated with endocrine organs and functions, such as insulin sensitivity. Meanwhile, brown fat comprises multilocular lipid droplets and mitochondria containing protein-1 (UCP1) [23].
Apart from white fat and brown fat, another type is beige or brite adipocytes (brown-in-white). These beige adipocytes are fat cells with a white fat phenotype, but stimulation can give a brown fat phenotype. This phenomenon is known as browning. This brown fat phenotype is associated with the function of increasing thermogenesis and preventing fat accumulation in the body, so it plays a natural anti-obesity role [24-26]. Browning is the appearance of beige adipocytes in white adipose tissue. This process increases thermogenic and exercise requirements. Various transcription factors are involved in this process, including the 16-containing PR domain (PRDM16), peroxisome proliferator-activated receptor (PPAR), and uncoupling protein (UCP) [24, 27].
Several factors can induce the browning process, such as cold exposure and a physiological stimulus. Previous studies have shown that cold exposure in rodents stimulates BAT signaling and increases UCP1 expression [28, 29]. Several compounds contained in food have been shown to have the effect of increasing the browning process [30]. Many animal and human studies have shown that exercise can induce adipose browning [31-34]. How the exercise mechanism induces browning remains unclear. One of the factors that may have a role in this process is the gut microbiome. Currently, the gut microbiome has been widely investigated because it has a vital role in the health of the human body, including its ability to induce browning in adipose tissue [35-37].
3. EXERCISE CHANGING GUT MICROBIOME PROFILE
Most microbiota are found in the intestine, with a density of up to 1013-1014 cells/gram of colonic mass [38, 39]. Gut microbiota have a variety of physiological functions, including digestion, metabolism, and the regulation of the immune system [39]. The human gut microbiota is mostly 90% occupied by the phyla Bacteroidetes and Firmicutes, and to a lesser extent, the phyla Proteobacteria, Actinobacteria, and Verrucomicrobia [40]. This composition can be influenced by various factors, including genetic factors, age, eating habits, disease conditions, and physical activity (exercise).
Exercise can modify the gut microbiome profile. Prior research has shown that exercise can reduce the ratio of Firmicutes to bateroidetes. The risk of obesity is associated with an increased ratio of Firmicutes to Bacteroidetes, which is linked to increased absorption of calories from the gut [41, 42]. Exercise can increase the colony of bacteria that produce butyrate, which is beneficial for health [18].
During exercise, various processes and changes occur in the body, one of which is in the digestive tract environment. Exercise can affect the intestinal transit time and splanchnic blood flow, the HPA axis, and increase urease-producing bacteria and mitochondrial function. These processes further affect the gut microbiome profile, including increasing the phylum Bacteroidetes, Verrucomicrobia, and Actinobacteria [43]. This mechanism relies on the exercise modality (type, intensity, and duration) [43].
3.1. Verrucomicrobia
The gut microbiome regulates metabolic conditions by several mechanisms, including maintaining intestinal barrier homeostasis, glucose homeostasis, and energy absorption [44]. Akkermansia municiphila is a species that belongs to the phylum Verrucomicrobia. Akkermansia has benefits for human health. It has the effect of increasing lipid oxidation and then increasing energy expenditure. Akkermansia municiphila is also important in the metabolism and degradation of mucin, thereby maintaining the integrity and homeostasis of the intestinal barrier [44, 45]. Yoon et al. showed an increase in the concentration of GLP-1 in high-fat diet (HFD) rats given A. municiphila. It was observed that one of the metabolic effects of A. munichipila comes from the regulation of appetite-regulating hormones [46]. Deppommier et al. found an increase in energy expenditure in high-fat diet (HFD) rats given pasteurized A. munichipila [47]. In addition, there was an increase in CO2 production and an increase in VO2 consumption with the administration of pasteurized A. municiphila in HFD mice [47]. This finding is supported by the results of Deng et al., which showed that increased levels of mRNA Cidea, Ppargc1a, and Prdm16, are markers of adipose browning in HFD rats given Akkermancia municiphila supplementation [37]. Various results of this study indicate the role of A. municiphila in inducing browning.
3.2. Actinobacteria
Actinobacteria (Bifidobacteria) is among the dominant phylum in the adult gut microbiome. Although the proportion is not too large compared to other phyla, Bifidobacteria have an important role in health and obesity prevention [48]. Phylum Actinobacteria plays a role in maintaining the intestinal barrier, starch degradation, and modulating the immune response by regulating regulatory T cells [49]. Fat absorption has been suppressed by Bifidobacteria supplementation. Therefore, it has the ability to inhibit an obesity-induced high-fat diet [50, 51]. This anti-obesity effect is also caused by the role of hormone GLP-1, which is an appetite-suppressant hormone. The gut microbiome profile can affect GLP-1 levels [50]. Similar findings by Cano et al. found that Bifidobacterium supplementation reversed increases in body weight and leptin levels in HFD-induced mice [52]. Infants who lack a Bifidobacteria population have a higher risk of obesity, diabetes and metabolic disease [48, 53, 54]. In addition, the supplementation of Bifidobacteria and lactobacillus can increase pro-inflammatory cytokines, such as IL-4 and IL-10. This pro-inflammatory cytokine increase further activates M2 and increases PGC-1α [55].
3.3. Bacteroidetes
One component of SCFA, namely propionate, has been shown to increase the production of hormones, Glucagon-like peptide-1 (GLP-1) and Peptide YY (PYY). Both of these hormones have the effect of suppressing appetite [41, 42]. The increase in butyrate is proportional to the relative abundance of Prevotella (Bacteroidetes) and Paraprevotella [56]. These Gram-negative anaerobic bacteria function in the degradation of proteins and carbohydrates. Diet rich in fiber is thought to be a factor that can increase the abundance of Prevotella [57, 58]. Prevotella can synthesize SCFA, which is the final product of fermented dietary fiber of intestinal microbiota. This synthesis process occurs through the acetyl-CoA pathway involving pyruvate as a substrate. SCFA has an important role in the body's metabolism. SCFA increases lipid oxidation and reduces white fat accumulation in adipose tissue, but the specific mechanism of SCFA in lipid metabolism is still being explored [59]. This mechanism is probably due to SCFA increasing the phosphorylation of PGC-1α and AMPK to increase lipid oxidation in tissues [60]. PGC-1α is one of the primary regulators of mitochondrial biogenesis and thermogenesis. An increase in PGC-1 and AMPK can further increase the impact of thermogenesis by increasing Ucp1. The relationship between exercise, gut microbiome profile, and its effect on adipose browning, is illustrated in Fig. (1).
4. GUT MICROBIOME METABOLITES INDUCE BROWNING
Changes in gut microbiome composition can affect energy metabolism. Prior studies have shown that exercise can increase the production of butyrate (SCFA) by gut microbiota [61]. Research by Gao et al. demonstrated the impact of butyrate in increasing the expression of the peroxisome proliferator-activated receptor-gamma coactivator 1-alpha (PGC-1α) gene, which is a regulator of mitochondrial browning and biogenesis [62]. Butyrate also increases respiratory capacity, fatty acid oxidation, and the activation of AMPK to reduce fat mass [63, 64].
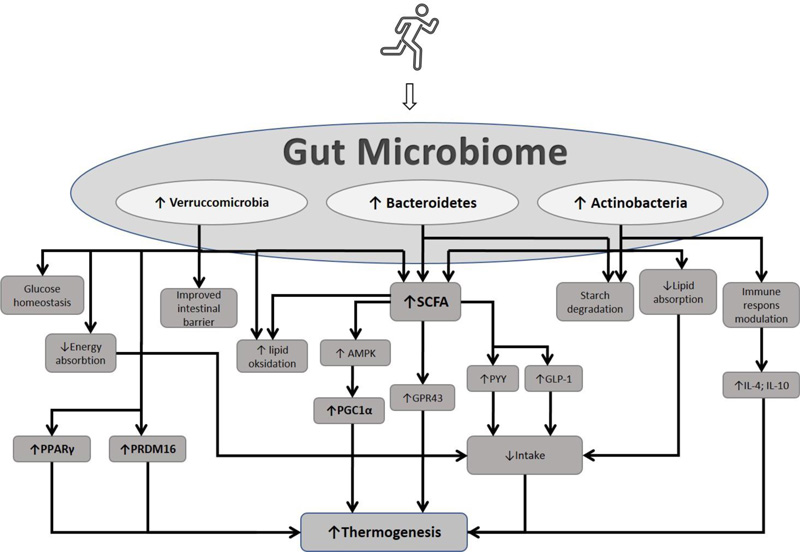
Li et al. reported an increase in Ucp1 expression accompanied by a decrease in lipid droplet size in butyrate-treated HFD mice [65]. SCFA can bind to its receptors in various body tissues, one of which is adipose tissue. Lu et al. reported that the supplementation of acetate, propionate, butyrate (Component SCFA), or a mixture thereof was shown to increase G-protein coupled receptor 43 (GPR43) expression in the adipose tissue of HFD-induced mice. The administration of this SCFA component also increased PYY and GLP-1 mRNA expression in the colon of HFD-treated mice [66]. SCFA supplementation also ameliorated the decreased expression of mitochondrial biogenesis genes (PGC-1a, NRF-1, Tfam) and beiging markers (Tbx1, Tmem26, CD137) in HFD-induced mice [66]. Exercise and/or butyrate supplementation have been shown to reduce elevated serum levels of lipopolysaccharide (LPS) and pro-inflammatory cytokines (TNF-a and IL-1ß) in HFD mice. In HFD-induced mice, lipid synthesis protein expression was significantly increased (ACC, p-ACC-Ser79, FAS). In contrast, there was a decrease in the expression of lipolytic proteins (CPT1, PPARa, PPARγ) in the liver of mice. Interestingly, these conditions could be reversed by exercise or the administration of butyrate [18]. This suggests that exercise promotes the browning of white adipose tissue, partly by modulating gut microbiome balance and increasing SCFA production. However, future studies are required to investigate the direct action of exercise on the browning of white adipose tissue. Various studies on the effects of gut microbiome and its metabolites on lipid metabolism and adipose browning are summarized in Table 1.
Year | Author | Method | Intervention | Importance |
---|---|---|---|---|
2017 | Li et al. [65] | In vivo | Intragastric and intravenous administration of butyrate Group: 1). HFD • without butyrate • with 5% butyrate intragastric • with butyrate IV 2). Vagotomy sub diafragmatik. One week after surgery, given treatment: - HFD - HFD with 5% butyrate for 5 weeks |
In Butyrate group: • ↓food intake; ↓ Body Weight; ↓ fat mass; ↓gWAT weight; ↓liver weight; ↓hepatic lipid content; • Plasma (↓ trigliseride; ↓fasting insulin; ↓ HOMA-IR • Adipose (↓Lipid droplet; ↑Ucp-1 expression; ↑TH (Tyrosine Hydroxylase)) • Gut: ↓ abundance of phylum Firmicutes relative to Bacteroidetes |
2016 | Lu et al. [66] | In vivo | 3-4 week-old C57BL/6 J male mice Group: • a control high-fat diet (HFD) • high-fat diet + acetate (HFD-A) • high-fat diet + propionate (HFD-P) • high-fat diet + butyrate (HFD-B) • high-fat diet + admixture (HFD-SCFA) |
• HFD+A/P/B or HFD+SCFA → suppressed weight gain • HFD+A/P/B or HFD+SCFA→ suppressed triglycerides, cholesterol, IL-1β, IL-6 or MCP-1 • HFD+A/P/B or HFD+SCFA → ↑ GPR43 level; ↓GLP-1 mRNA in adipose and colon • HFD+A or HFD+SCFA →↓leptin mRNA, ↑ adiponectin and resistin mRNA • HFD+P →↓PYY mRNA expression • Cpt1c and cpt2 expressions have a positive relationship with GPR43 in adipose. • HFD+SCFA → ↑ PGC-1a, NRF-1, Tfam, -F1-ATPase, COX IV and cyt-c); mtDNA levels, Tbx1, Tmem26, CD137 • HFD+A/P/B or HFD+SCFA → ↑rich bacterial phylotype in the gut. |
2009 | Gao et al. [62] | In vivo | 4 weeks old, male C57BL/with HFD HFD for 13 weeks |
• Butyrate group: • ↑expenditure on HFD; ↑oxygen consumption at night; ↑Substrate use (assessed by RER) ; suppressed ↑BW; suppressed ↑ body fat content; • Plasma: ↓ Fasting glucose ; ↓Fasting insulin; ↓HOMA-IR; ↓Intraperitoneal insulin tolerance • Adipose: ↑ smaller lipid droplets; ↑ PGC-1α; ↑UCP1 expression (RT-PCR and immunoblot) |
2021 | Yu et al. [18] | In vivo | 4-weeks-old C57BL/6 male mice Group: • NCD (sedentary control group (NC) or exercise training group (NE) for 8 weeks. • HFD group: a) HFD control group (HC); b) HC + oral sodium butyrate (HCB); c) HFD + exercise training (HE); d) HE + oral sodium butyrate g (HEB) for 8 weeks. |
• HCB, HE, HEB → suppressed ↑ energy intake • HE →↓plasma triglycerides (TG), total cholesterol (TC), FFA levels • HCB →↓serum TG and TC • HE & HEB → suppressed ↑LPS & pro-inflammatory cytokines (TNF-a & IL-1ß) • HE → ↑ fecal butyrate; ↑ level phylum Bacteroidetes & Firmicutes • HE, HCB → ↑ SESN2 and CRTC2 expression • HCB → suppressed ↑ IL-1β and TNF ɑ |
2019 | Li et al. [67] | In vivo | Rats were grouped into: • Wild type (Control) C57BL/6J 7-8 weeks old • Germ-free male mice (GF) aged 11-12 weeks (given antibiotics) ABX • Pairpad Antibiotic treatment is started in 8-9 weeks old animals for 3-4 weeks |
ABX group → ↓ thermoregulatory effect; ↓ mRNA Ucp1& ↓ Ucp1 protein (cold exposure); ↓ oxygen consumption (VO2); ↓ Eosinophils in BAT; ↓Fecal butyrate Treatment CL316243 • ↑multilocular lipid droplets adipocytes in WAT rats SPF vs. GF • ↑Ucp1 protein and Ucp1 mRNA • Mice with repopulation microbiota (RO)→ ↑Ucp1 (cold exposure) Treatment butyrate: • ↑Core body temperature ABX • ↑Ucp1 protein in BAT & WAT • ↓food intake of control rats, but not in ABX. • ↑ fecal butyrate. |
2020 | Deng et al. [37] | In vivo | Male C57BL/6 mice were randomly divided into 6 groups, including: - HFD + A.muciniphila I - HFD + A.muciniphila II - HFD + A.muciniphila PBS - NCD+ A.muciniphila I - NCD+ A.muciniphila II - NCD+ A.muciniphila PBS |
A. muciniphila group: • Plasma → ↓fasting blood glucose; ↑serum insulin; ↓serum levels of TG and TC • Liver → Suppressed ↑ Liver weight; suppressed lipid drop accumulation • WAT → (Amuc_GP01 and Amuc_GP25) ↓adipocyte dimension • BAT → ↓BAT whitening (changed from multilocular to unilocular adipocyte); ↑UCP1 protein and mRNA UCP1 expression; ↑mRNA levels of Cidea, Ppargc1a, Prdm16 and Pparg • ↓mRNA expression of macrophage inflammation markers |
2020 | Depommier et al., [47] | In vivo | 14 C57BL/6J 8-weeks old male mice Groups; ND or an HF; daily oral intervention with pasteurized A. muciniphila was given. |
Pasteurized A. municiphila group: • ↓weight gain; ↓ fat mass gain • ↓Adipose depots (SAT: subcutaneous; EAT: epididymis; VAT: visceral) • ↑ energy expenditure; ↑CO2 production; ↑ VO2 consumption • ↓whitening and lipid droplet size of BAT • |
2021 | Yoon et al. [46] | In vivo |
HFD-induced C57BL/6J mice Identification and analysis of P9 protein secreted by L cells of HFD mice were carried out using spectrophotometry |
Municiphila group: • ↓body mass; ↑ glucose tolerance; ↑serum concentrations of markers of insulin and oxidation genes. • ↑mitochondria-specific gene encoding Ucp1 • ↑ number of anti-inflammatory M2 macrophages in iBAT • ↑ plasma GLP-1 concentrations • ↑ the expression of Gcg (the gene encoding GLP-1) and Pcsk. |
CONCLUSION
Exercise can induce adipose browning, possibly by modifying the gut microbiome profile. Exercise increases the butyrate-producing bacteria, including phylum Verrucomicroia, Bacteroidetes and Actinnobacteria. This increase in phyla increases the gut barrier, maintains glucose homeostasis, modulates the immune system, reduces intestinal energy and lipid absorption, increases lipid oxidation, increases GLP-1 hormone, and increases an important gut microbiota metabolite, namely, SCFA. Finally, we conclude that the set of mechanisms synergistically enhances the pathways that induce adipose browning.
FUTURE PERSPECTIVE
Studies on the effects of exercise on the gut microbiota and its metabolites and on adipose browning are still not widely available. Therefore, we plan to further explore the primary effect of exercise on the gut microbiome and adipose browning.
LIST OF ABBREVIATIONS
SCFAs | = Short-chain fatty acids |
WAT | = White fat tissue |
BAT | = Brown fat tissue |
PPAR | = peroxisome proliferator-activated receptor |
HFD | = High-fat diet |
CONSENT FOR PUBLICATION
Not applicable.
FUNDING
This study was funded by Internal Research Grant UNPAD no. 2203/UN6.3.1/PT.00/2022 to HG and VMT.
CONFLICT OF INTEREST
The authors declare no conflict of interest, financial or otherwise.
ACKNOWLEDGEMENTS
Declared none.