All published articles of this journal are available on ScienceDirect.
Use of the Testosterone/Cortisol Ratio Variable in Sports
Abstract
This critical review discusses the use of the testosterone/cortisol ratio in the studies of athletic performance and sports physiology. Although in most of the time physical exercise is beneficial to health, it can also be seen as a “stressor” both in men and in women. It is not completely known at what level this “physical stress” ends up its beneficial effects and begins to impair health status. In search for this putative turning point, several markers have been put forward in the last decades. One of these markers is the ratio between testosterone, considered as an anabolic hormone, and cortisol, considered as a catabolic one. Whether in search for an anabolic internal environment for strength training or to avoid performance decline during aerobic workout, the testosterone/cortisol ratio has been considered as an important physiological variable to gauge individual conditioning and responses.
INTRODUCTION
It has been established that physical activity promotes heath [1]. Although physical activity and physical exercise are beneficial most of the time, it can also be seen as a “stress factor” in men and women. Despite the difficulties of defining the concept of stress on the human and other animals, in general physical stress can be classified as acute or chronic, and hormones such as testosterone and cortisol can be used to assess the metabolic alterations caused by exercise [2].
In sports physiology, the testosterone/cortisol ratio has been used to analyze the balance between anabolic and catabolic processes. Since testosterone shows anabolic effects and cortisol promotes catabolic effects, the testosterone/cortisol ratio has been considered as a marker of overreaching and overtraining syndromes (states in which individuals experience a decline in performance, psychological changes, and neuroendocrine disorders following certain physical training). In these states, a catabolic internal environment would be present, associated with certain chronic stress caused by exercise.
Almost thirty years ago, Adlercreutz et al. [3] suggested that a decline greater than 30% in the resting plasma testosterone/cortisol ratio would be indicative of overtraining. Although important as an overall approach, recently such a view was criticized because it was observed that a greater than 30% decline in testosterone/cortisol ratio did not always result in a deterioration of athletic performance [4]. Anyway, in the absence of other variables, researchers continue to consider the testosterone/cortisol ratio as an useful guideline in the evaluation of acute and chronic effects of athletic training, both in strength training and in endurance training.
Produced primarily in testes, testosterone is a hormone that is present in greater quantities in males. In females, this hormone is produced by the adrenal glands, the ovaries, as well as through peripheral conversion of circulating androstenedione. Despite the testosterone concentrations in women are about one-tenth the concentrations in man, the response of testosterone to acute physical exercise seems to be of increment. In untrained men, both the strength exercise and the endurance exercise, increase total and free testosterone after about 15-20 minutes of activity [5]. The cortisol response appears to be similar in both sexes, increasing in response to moderate exercise both short and long term.
When considering acute responses, most fleeting hormonal changes, such as a greater than 30% drop in testosterone/cortisol ratio, occur in amateur male athletes after marathon races, but such hormonal changes tend to become normalized in the following days of the strenuous exercise and do not correlate with the signs and symptoms of overtraining. Such a response may vary depending on the fitness of the athlete. During a chronic response, the hormonal levels may “remain altered” for prolonged periods and can serve as a variable for the broader analysis of physical training.
In terms of medical aspects, low testosterone levels may be indicative of poor health in men. Extreme levels of circulating androgens, whether high or low can have negative effects on women’s health. In a population-based prospective study of chronic stress-related heart disease in men, high cortisol to testosterone ratio showed strong positive associations with the components of insulin resistance syndrome [6]. In women, the chronic hypercortisolism was found to be associated with isolated exercise-induced amenorrhea. Results from the analysis of bone mineral density changed the idea that athletic amenorrhea was a benign event and linked this phenomenon to premature bone loss-associated declines in the levels of progesterone and estradiol [7]. Also, some diseases such as anorexia nervosa and severe depression have also been found to be associated with chronic hypercortisolism [8]. The possible chronic hypercortisolism caused by sports activity in elite athletes, and its effects on long-term health, particularly in man, is not well studied.
Testosterone/Cortisol Ratio and the Stress System
The involvement of a complex regulatory network, which includes biological clocks that control circadian rhythms of hormonal secretions, renders the analysis of hormonal effects difficult, and even the analysis of seasonal rhythms is challenging [9].
Cortisol, the main form of glucocorticoid in humans, is a catabolic hormone secreted by the adrenal cortex in response to physical and psychological stress. Exercises which require 60% or more of the maximum oxygen consumption of an individual (VO2 max), are one of the physical stressors that could cause increased cortisol secretion [10, 11]. Cortisol affects metabolism by contributing to the maintenance of blood glucose levels during exercise. Cortisol does this by acting on the skeletal muscle and the adipose tissue to increase the mobilization of amino acids and lipids as well as by stimulating gluconeogenesis [12, 13]. While cortisol levels increase during exercise, most of the changes and effects of this hormone occur during the early recovery period after the exercise bout [14]
Testosterone is considered as an anabolic hormone with several key physiological functions in humans. In males, testosterone is primarily produced and secreted by Leydig cells of the testes. Testosterone and its active metabolite, dihydrotestosterone-DHT, are the most important androgens and exert biological effects through their free portions. Testosterone is primarily inactivated in the liver and is excreted through feces or urine. In females, the primary sources of androgens originating from the cholesterol are the adrenal glands, ovaries, and peripheral tissues such as adipose tissue, muscle, and skin. Kirschner et al. [15] reported that in young women, the sources of testosterone include ovary (25%) and adrenals (25%), and that it is also produced through peripheral conversion (50%). Testosterone is important for the growth and maintenance of skeletal muscle, bone, and red blood cells [16].
Physical exercise can be seen as a stress model, where some internal or external stressors may alter the organism’s dynamic equilibrium or homeostasis. Physical and behavioral changes occur as a response to these stressors. Once a threshold is reached, the stress system elicits systemic responses in the brain and in the peripheral elements as the autonomic sympathetic and the hypothalamic–pituitary–adrenal system. Selye [17] proposed the term “normal stress”, and in the case of sports, this normal response could stimulates bodily functions and could generate hormonal responses through multiple pathways, helping for example to the maintenance of blood glucose levels during an acute exercise practice, as said above. The harmful stress, however, is not well defined in the case of sports. Some questions can be made: In an acute point of view, an important drop in testosterone/cortisol ratio as seen after marathon races, can indicate a “normal stress” or a harmful stress? In a chronic point of view, the inhibition of hypothalamic –pituitary–gonadal axis in the case of isolated exercise-induced amenorrhea could indicate a harmful stress?
The vasopressin (AVP) and the corticotropin-releasing hormone (CRH) neurons in the paraventricular nucleus of the hypothalamus (PVN) and the locus ceruleus (LC)/norepinephrine (NE)-sympathetic system neurons in the brainstem are the central commanders of this stress system [2]. Intense physical exercise stimulates the HPA and HPG axes through several mechanisms that are not fully understood. Increase in plasma lactate and humoral mediators such as interleukins and angiotensin II have been implicated in the activation of the HPA axis during intense physical exercise [18]. The beta-endorphins, factors released into the circulation during intense exercise, influence the hypothalamic functions, including the regulation of reproduction via their inhibitory effect on GnRH release [19]. The endogenous opioids (beta-endorphin) and ACTH are derived from proopiomelanocortin (POMC) and corticotropin releasing hormone (CRH) stimulates the secretion of these factors [20]. CRH such as opioids inhibits the hypothalamic –pituitary–gonadal axis, and physical stress stimulates the release of these factors [21].
The interaction of the hypothalamic–pituitary–adrenal axis and hypothalamic–pituitary–gonadal axis is complex (Fig. 1) and differs in males and females. Fig. (1) shows this interaction of the hypothalamic–pituitary–adrenal axis and hypothalamic–pituitary–gonadal axis supported by studies done in animals and humans. A deep investigation of this interaction was conducted in females because the isolated exercise-induced amenorrhea.
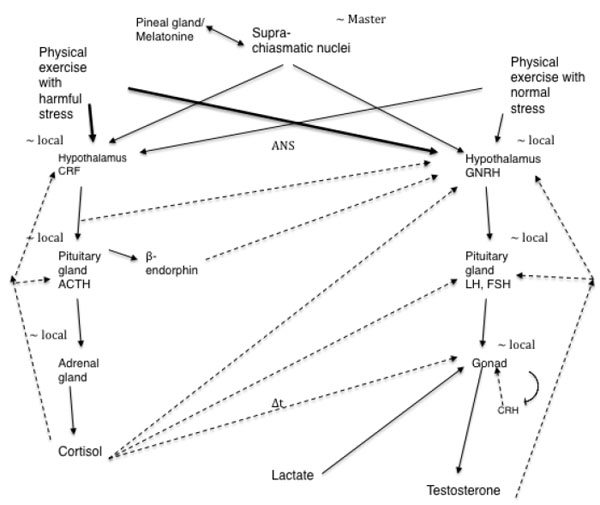
Lu et al. [22] suggested that the increase in plasma testosterone levels observed in male rats during exercise is at least partially due to direct stimulation and LH-independent effect of lactate on testosterone secretion in the testis. This is mediated by the elevated levels of cyclic adenosine monophosphate (cAMP) in these organs. Generally, an increase in plasma testosterone levels is followed by gonadotropin stimulation. However, studies have shown that LH levels remain unchanged after exercise [23-25]. In contrast, Cumming et al. [26] found increase in LH after exercise. Kraemer [27] measured the serum testosterone levels before, during, and after heavy exercise and found that circulating testosterone levels significantly increased in men, but not in women. Thus, the mechanisms underlying the exercise-induced increase in testosterone levels remain unknown.
Direct stimulation by catecholaminergic agents resulted in testosterone production in some studies, what could explain the rapid rise in testosterone levels in response to various stimuli. An in vitro study using immature testis of the golden hamster found that catecholamines, acting through both alpha- and beta-adrenergic (norepinephrine and epinephrine) receptors, are potent physiological stimulators of testosterone production [28]. In a study of healthy men subjected to bicycle ergometer exercise, Ježová and Vigaš [29] found that blockade of beta-receptors by propranolol inhibited the testosterone response to exercise. Conversely, using the immobilization stress model in rats, the sharp decline in serum testosterone is one of the first signs found in some studies. This reduction in testosterone production induced by acute stress appears to be related to inhibition of the activity of testicular steroidogenic enzymes [30, 31]. On these last studies mentioned, there seems to be a divergent response of testosterone in acute stress model of immobilization in rats compared to acute physical stress model in humans.
Similar to cortisol, testosterone levels increases linearly in response to physical exercise until a specific threshold of exercise intensity is reached, with peak concentrations generally occurring at the end of physical activity [32]. However, some studies have shown that there is a negative relationship between cortisol and testosterone under specific conditions.
Bambino and Hsueh [33] demonstrated a direct inhibitory effect of high doses of glucocorticoids on the function of the Leydig cells of the testes in mice, where such doses attenuated testosterone production in a dose and time-dependent manner. Further, these authors found that glucocorticoids decreased the amount of testicular LH receptors. Cumming et al. [34] found a similar relationship in humans. These investigators speculated that cortisol could interrupt the testicular testosterone production by disrupting the biosynthesis of the hormone.
Rivier et al. [35] suggested that the response of the pituitary-testicular system to stress is biphasic, with an initial stimulatory phase and a subsequent inhibitory phase that is prolonged if the stress is of sufficient magnitude. The authors also suggested that most immediate changes in LH secretion are mediated at the level of GnRH-secreting neurons, whereas long-term effects also involve peripheral mechanisms such as alterations in the response sensitivity of the pituitary and the gonads. Hoffman et al. [36] also found this behavior of total testosterone in response to submaximal exercise of different durations. Exercises lasting up to 2 hours and 30 minutes resulted in elevated testosterone levels. After this period, testosterone levels decreased, more rapidly in cases where exercise lasted for 4 hours (∆t in Fig. 1 linking cortisol to the gonad represents a delay in the cortisol action of counterbalancing the production of testosterone).
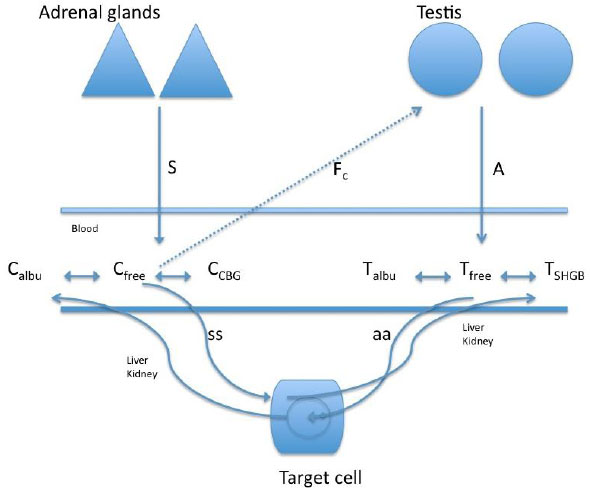
In a study of the relationship between cortisol and testosterone in men (n = 45, 26.3 ± 3.8 years) at rest and after exercise, 30 participants practiced 60-minutes race (70 to 75% of max V02), 12 bicycled for 90 minutes (65% of max V02), and 3 practiced paddle for 60 minutes (65% of max V02) [37]. The authors found a negative relationship between the total testosterone and cortisol after exercise, whereas a positive relationship between cortisol and free testosterone was observed after exercise. These authors suggested that the increase in free testosterone after exercise was likely due to the contribution of the adrenal cortex or the dissociation of free testosterone from the sex hormone binding globulin. However, considering such possible biphasic response of testosterone, the duration of the exercise in this study may have been short for the response of free testosterone to decrease more sharply (Fig. 2 shows the interaction of the free portion of the hormones with the binding proteins).
In vitro studies have shown that cultured rat Leydig cells secrete CRH into the culture media [39, 40]. In addition to its negative effects on the central hormonal axis, CRH, a key neuropeptide involved in the stress responses, exerts an inhibitory effect on the testis, acting through the cell surface receptors on Leydig cells, being a negative regulator of LH, inhibiting the gonadotropin action and testosterone production.
However, in rats, immobilization stress reduces the plasma testosterone without affecting the plasma LH concentrations [41, 42]. A study that investigated the effect of immobilization stress (immobilization of 3 hours) on testicular steroidogenesis found an 82% reduction in testosterone levels and significantly elevated levels of plasma corticosterone [43]. This immobilization stress inhibited the activity of 17 alpha-hydroxylase and 17, 20-lyase without affecting the binding of LH/hCG receptors. This inhibition could explain the reduced testosterone levels (Fig. 1). Here it is important to say that immobilization stress causes physical and psychological stress.
Several studies have shown increased cortisol responses with concomitant decrease in testosterone in groups of amateur male runners who participated in marathons [44-46]. Such divergent relationship between the two hormones appeared to be restricted to a period of transitory hypercortisolism of few hours. In these groups of marathon runners, the reduction in testosterone levels occurred approximately after 4 hours of relative hypercortisolism due to a total physical exercise. Since it is speculated that cortisol may have a direct action that reduces the testosterone production in the testes, such a decline could be observed after a marathon race in male amateur runners. This could show the proposed biphasic response of the pituitary–testicular system to stressful stimuli [35]. At first, testosterone may rise with the initial stress of a marathon. After a period, cortisol, which also rises in the blood, may counteract the testicular synthesis of testosterone.
However, this type of acute biphasic response appears to be related to the extent of activation of the hormonal axis. Studies have shown that in long distance runners, the acute response is markedly reduced compared to that in middle-distance runners [47]. In a study that evaluated the hormonal responses of a group of professionals and amateur male runners during endurance training, it was observed that there was an increase in cortisol two days before the marathon only in the group of amateur runners, whereas anabolic effects were observed in professionals [48]. This might be due to better physical conditioning, which may be associated with reduced pituitary–adrenal activation in response to the training volume [49]. Similarly to that in chronic users of glucocorticoids, an investigation of the effects of chronic hypercortisolism in highly trained athletes revealed downregulation of the alpha isoform of the glucocorticoid receptor in peripheral blood mononuclear cells [50].
Testosterone/Cortisol Ratio in Strength Training and Endurance Training
The testosterone/cortisol ratio has been studied extensively in sports physiology. During strength training, an anabolic environment promotes protein synthesis and muscle hypertrophy (muscle fibers type IIb). The anabolism/catabolism ratio can be monitored also in endurance training for improving the aerobic metabolism of muscles (muscle fibers type I). Both in strength training and in endurance training, attention is paid to the signs and symptoms of overtraining.
Ahtiainen et al. [51] found a significant correlation between the increase of testosterone caused by physical training and the muscle cross-sectional area, suggesting that acute increases in testosterone may play an important role in muscle hypertrophy. However, acute responses are blunted in women and the elderly, reducing the hypertrophic potential in these populations [52-54]. Both total and free testosterone levels have been used for evaluating the training volume in men and women [55]. For example, Vervoorn [56] suggested that unlike that observed in professional male rowers the free testosterone/free cortisol ratio is not a good indicator of anabolic/catabolic balance in professional female rowers.
West and Phillips [57] investigated the associations between acute exercise-induced hormonal responses and the adaptations to high-intensity resistance training in a cohort of 56 young men. Alterations in the growth hormone (GH), free testosterone, IGF-1, and cortisol were measured at the midpoint of a 12-week training program and were correlated with marking variables of training adjustments, including lean body mass, muscle fiber cross-sectional area, and leg press strength. Rises in GH were positively correlated with the changes in type I fiber in the muscle cross-sectional area. Cortisol and GH, but not testosterone and IGF-1, were positively correlated with the increases in type II fiber area. Rises in cortisol were positively correlated with change in whole-body lean body mass. No hormones were associated with the change in the leg press strength. These researchers suggested that an internal environment with high levels of “anabolic hormones” need not be associated with a gain of muscle or gain of strength. The rises in testosterone after a strength training, for instance, could produce a fleeting physical stress response not correlated with hypertrophic gains [57-59].
Thus, despite the anabolic hormonal responses to physical exercise were not correlated with gains in muscle fiber in some well-designed studies [57, 58], some reports have shown association of testosterone with motivation and readiness to compete or perform, as well as improved acute neuromuscular performance in athletic populations [60, 61].
The most current consensus on overtraining says that testosterone/cortisol ratio decreases in relation to the duration and intensity of training and this ratio indicates only the tangible physiological strain of training. The ratio cannot be used for diagnosis of overreaching and overtraining syndromes [4], however, these syndromes could be associated with maladaptation of the hypothalamic pituitary adrenal axis and other hypothalamic axes. Some studies put forward the idea that overtraining is the end of a process that initiates with a disturbance, progresses to an adjustment, and ultimately ends up as a maladaptation of all hypothalamic axes.
The possible hypercortisolism due to sport training could indicate a condition with negative effects on health. However, some studies did not observe these effects related to 24-h urinary free cortisol excretion and morning plasma cortisol concentrations in resting endurance-trained men compared with age-matched sedentary subjects [9, 62, 63]. This brings the doubt if the men well trained to some modalities of sports can develop a chronic hypercortisolism. Luger [49] observed that highly trained runners showed minor responses of cortisol due to exercise as compared with other two groups. Trained runners presented mild hypercortisolism while in basal conditions and, during exercise, they had milder responses of the HPA axis [2, 49]. Smith [6] associated the general concept of stress to the relationship between cortisol and testosterone and found that the high cortisol to testosterone ratio showed strong positive associations with the components of insulin resistance syndrome. Nevertheless, the association of the stress caused by physical exercise with general health outcomes, especially in men, is an issue that still needs to be deepened.
CONCLUSION
Identification of markers that can be used to evaluate the stress caused by physical exercise is important to human health. Testosterone/cortisol ratio is variable currently used in the evaluation of the organism response to acute and chronic physical stressors. The interpretation of these data collected from the athletes is challenging. The relationship between the testosterone/cortisol ratio and the anabolism/catabolism balance needs to be further clarified in terms of strength training. Nevertheless, researchers and practitioners in the filed of sport physiology still use the testosterone/ cortisol ratio in the evaluation of the intensity of training.
The interaction between the hypothalamic–pituitary–adrenal axis and the hypothalamic–pituitary–gonadal axis is complex and may be different in males and females. Studies have shown increased cortisol responses with concomitant decrease in testosterone in groups of amateur male marathon runners, which might be caused by the direct action of cortisol that reduces the testosterone production in the testes. This acute dynamic response of the T / C ratio still need to be exploited, with respect to the personal responses of each individual, and with respect to the connection of these hormones with their binding proteins.
The chronic hypercortisolism caused by sports and its consequences for the long-term health of male athletes is not well studied. In women, studies have shown that chronic hypercortisolism associated with amenorrhea induced by exercise have a great impact on health, possible from interaction between the hypothalamic–pituitary–adrenal axis and the hypothalamic–pituitary–gonadal axis.
CONFLICT OF INTEREST
The author confirms that this article content has no conflict of interest.
ACKNOWLEDGEMENTS
Declared none.