Biomechanical Analysis of an Overhead Baseball Throwing Movement Associated with a Cardiorespiratory Fatigue Effect
Abstract
Background:
Overhand pitching is a movement that requires the coordination of lower extremity, trunk, and upper extremity segments to effectively transfer force throughout the kinetic chain to project a baseball.
Objective:
The purpose of this study was to investigate the effect of cardiorespiratory fatigue on throwing ball velocity related to kinematics motion changes in baseball.
Methods:
The study samples included 12 male baseball athletes (19.3 ± 2.8 years old, height of 1.71.4 ± 3.1 m, and weight of 64.3 ± 3.6 kg). A descriptive quantitative method approach was used. The instruments included two high-resolution handycams (Sony HXR-MC2500, Japan), a high-speed camera (Fastec Imaging TS5-H, USA), a radar speed gun (Bushnell 101922, Germany), a 3D force platform (The AMTI Optima Series 20210, USA), 14 point manual markers, a motion capture system software (Frame DIAZ IV, Japan), and a heart rate sensor (Polar H10, Finland).
Results:
The results of this study showed that there were significant differences in the ball velocity (p= 0.042), stride length percentage height (p= 0.041), elbow flexion (p= 0.046), maximum shoulder horizontal adduction (p= 0.041), maximum elbow extension angular velocity (p= 0.035), maximum shoulder internal rotation (p= 0.029), and lateral trunk tilt (p= 0.029) when the pitchers were fatigued.
Conclusion:
Cardiorespiratory fatigue causes changes in the kinetic harmonization of upper and lower body motion, which results in a decrease in the ball velocity. These results are similar to those in previous studies conducted with the intervention of muscle fatigue during overhead baseball throwing, which causes a decrease in the performance and ball velocity.
1. INTRODUCTION
Maintaining the physical condition of a pitcher during a tournament affects the pitching speed performance because the 1 m·s−1 increase in the speed has an impact on the reaction time of the batter [1-3]. The pitcher quality depends on the hand muscle strength capacity and the perfect movement phase mechanism that involves six phases, i.e., wind-up, lead foot contact, arm cocking, arm acceleration, ball release phase, and follow-through [4]. Overhand pitching is a movement that requires the coordination of the lower extremity, trunk, and upper extremity segments to effectively transfer force throughout the kinetic chain to project a baseball. It has been reported that angular velocities at the shoulder joint during overhand pitching can reach magnitudes near 7000°/s at the point of ball release [5].
The initial motion to the follow through phase helps to transfer energy from the leg during the drive and improve energy after the contact of the leg. Effective pitching is determined by a complicated relationship between an increased body segment speed, starting from lower to upper body segments [6]. The accuracy and speed of pitching should be taken into account to prevent the opponent from hitting the ball [7]. According to Solomito, Garibay & Nissen (2018), pitching in baseball requires a series of complex movements because coordinated fast movements occur to provide an energy transfer from the lower leg, to pitching arm, and finally to the ball. Overhand pitching motion consists of a sequence of body movements starting from lifting the leg, moving the hip and trunk, and finishing with the upper body extremity movement to push the ball toward the home plate [8-10]. The pitching motion of a pitcher consists of a continuous sequence, which requires a dynamic power with the precise timing and coordination of body segments to produce maximum accuracy and speed [11]. Owing to the importance of the pitching motion phase and kinetics chains during pitching, a distraction of the body function will lead to weak pitching performance [12]. For a long time, the fatigue phenomenon has been of interest in different areas of sports medicine and sports performance. Strength and conditioning coaches implement training programs aimed at minimizing muscular fatigue and performance decrease [10-13].
At the onset of muscular fatigue, pitchers alter many kinematic (movement pattern) parameters of their pitching technique, including arm, trunk, and knee positions. Marsh et al. (2018) observed a considerable decrease in the pitching velocity, maximal shoulder external rotation, knee flexion, maximum shoulder distraction force, maximum elbow distraction force, horizontal abduction torque at ball release, and maximum horizontal abduction torque at the end of a five to six inning pitching games. When fatigued, pitchers had a decrease in the ball velocity of nearly 4.24 m·s−1, a decrease in the maximal shoulder external rotation by 9º degrees, and the stride leg landed with more knee flexion. An increase in the stride leg knee flexion and maximal shoulder external rotation upon landing may indicate a loss of coordination in pitching timing patterns that may affect ball velocity. Escamilla et al. (2006) showed that a pitcher begins to feel muscle fatigue at the 5th to 6th inning. During these innings, the pitching speed significantly decreases, which is caused by a decrease in the speed of maximal shoulder external rotation and a change in the angle of the knee joint when releasing the ball [12-17]. The average velocity of the ball reaches 40 m·s−1 at the first inning and gradually decreases during the game until only 24 m at the last inning [18, 19].
Furthermore, Okoroha et al. (2018) showed that under simulated conditions, the fatigue experienced by the pitcher increases after successive innings. Another study [20, 21] also examined kinematic changes when the pitchers became fatigued over several innings; these researchers observed an increase in the knee flexion during maximal glenohumeral external rotation and ball release and identified an increased hip lean during hand separation, which is also known as the stride of the pitch stride. These changes in upper and lower extremity kinematics may indicate a loss in coordination and timing between segments [1], which may affect the efficiency of force transfer from lower to the upper extremities and put the pitcher at risk of a performance decrease. In addition, fatigue is a factor that affects the quality of motion mechanics of a pitching motion technique as an effort to maintain the speed and accuracy of an ideal pitch [22]. These results suggest that an increase in the number of pitches by the pitcher induces muscle fatigue. Fatigue is a crucial problem in baseball, which has been extensively discussed in scientific studies [23-27]. Fatigue may be a factor that prevents and interrupts the decision-making process of the pitcher and hinders the cognition of the pitcher during the tournament [18-20].
On the basis of the above-mentioned introduction, it is possible to conclude that muscle fatigue produces a decrease in throwing performance with slower ball speeds owing to changes in body mechanics. Pitcher throw related to muscle fatigue has been widely studied. However, studies related to cardiorespiratory fatigue rarely analyze changes in ball throwing speed and pitcher motion kinematics. Therefore, the purpose of this study was to investigate the effect of cardiorespiratory fatigue on throwing ball velocity related to kinematics motion changes in baseball.
2. MATERIALS AND METHODS
2.1. Participants
The subjects of this study were university level male baseball players who were randomly chosen and included 12 pitchers with the mean aged 19.5 years old, height of 171.4 cm and weight of 64.3 kg. All 12 participants had over 5 years of experience playing team baseball. The subjects were fully informed about the protocol before participating in this study. Informed consent was obtained prior to all testing from the subjects. The study was conducted in accordance with the recommendations of the ethics committee of the Universitas Pendidikan Indonesia.
2.2. Instruments
The instruments used in this study were two high-resolution handycams (Sony HXR-MC2500, Japan), a high-speed camera (Fastec Imaging TS5-H, USA), a radar speed gun (Bushnell 101922, Germany), a 3D force platform (The AMTI Optima Series 20210, USA), a motion capture system software (Frame DIAZ IV, Japan) and a heart rate sensor (Polar H10, Finland).
Fig. (1) shows that 14 point manual markers were placed on the left and right shoulder joint, elbow joint, wrist joint, hip joint, knee joint, ankle joint, neck, and torso.
2.3. Procedure
To examine the pitcher pitching motion, three video cameras were used. The first camera was placed perpendicularly next to the pitcher at a 3 m distance. Camera 2 was placed perpendicularly in front of the pitcher at a 2 m of distance. Meanwhile, camera 3 was placed perpendicularly above the pitcher at a 1.5 m distance. The 3D force platform was placed in front of the pitcher’s foot at a 45 cm distance; the speed gun radar, which was used to measure the speed of the ball, was placed perpendicularly in front of the pitcher at a 4 m distance.
Fig. (2) shows that before the trial under unfatigued condition, the subjects were instructed to warm up for 15 min. After 5 min resting period, the subjects were asked to shoot the ball as fast as possible towards the entire goal area without a target to measure the speed of the ball as the optimum ball speed reached by the subjects. Throwing ball velocities were measured using a sports radar gun Bushnell 101922, Germany. The mean value of the 6 shots was calculated, and ball velocity was measured in m·s−1.
Then, all subjects performed a fatigue cardiorespiratory test on a treadmill. Before the treadmill test was carried out, all subjects were fitted with a heart rate monitoring system (Polar H10 Polar Electro OY, Finland). The treadmill speed started at 8 km·h−1 as a warm-up session, and continued at 9 km·h−1, and increased by 2 km·h−1 every 3 min, treadmill test was a continuous straight line running [20, 21]. The test was ended when the subjects were exhausted. Subsequently, all subjects were asked to continuously throw the ball 6 times as fast as possible towards the entire goal area. The mean velocity value of the 6 shots was calculated, and the ball velocity was measured in m·s−1.
2.4. Kinematics Parameter Analysis
Fig. (3) shows the 11 kinematic body segment and joint position parameters (including elbow flexion, shoulder external rotation, shoulder abduction, shoulder horizontal abduction, forward trunk tilt, pelvis rotation, upper trunk rotation, lead knee flexion, stride length, lead foot position, and lead foot) measured at the instant of lead foot contact.
In Fig. (3), the global X direction is represented by a vector from the pitching area towards the force platform; global Z is represented by a vector pointing vertically; global Y is the cross-product of Z and X. Stride length is defined as the linear distance between the platform and the lead ankle at lead foot contact. Front foot placement was represented as the distance between the rear ankle and the lead ankle at the lead foot contact in the global Y direction. Lead foot angle was represented as the angle between the longitudinal axis of the lead foot and the global X direction in the global XY plane.
Lead knee flexion is the angle between the distal directions of the thigh and shank vectors. The pelvis rotation angle is the angle between a vector drawn from the throwing side hip through the lead hip and the global X direction in the XY plane. The upper trunk rotation angle was the angle between a vector drawn through the upper trunk (throwing shoulder to lead shoulder) and a vector drawn through the hips in the XY plane. Contralateral trunk tilt is the angle between the superior direction of the trunk and the global Z direction in the YZ plane.
Trunk forward tilt is the angle between the superior direction of the trunk and the global Z direction in the XZ plane. Shoulder abduction is the angle between the distal direction of the upper arm vector and the inferior direction of the trunk in the trunk frontal plane. Shoulder horizontal abduction is the angle between the distal direction of the upper arm and an upper trunk vector in the transverse plane.
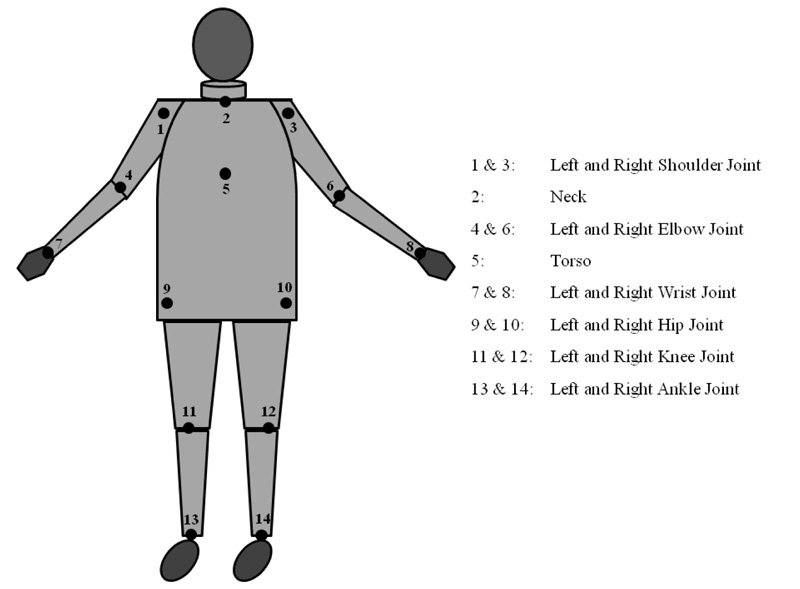
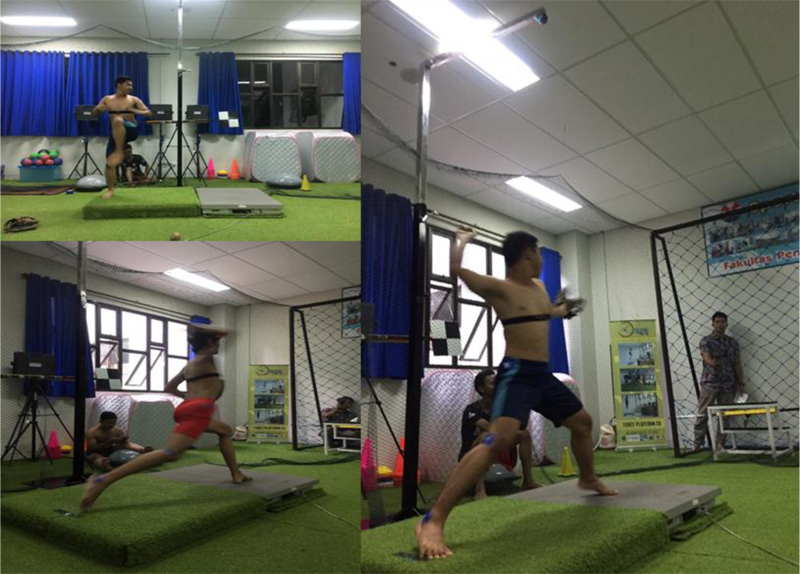
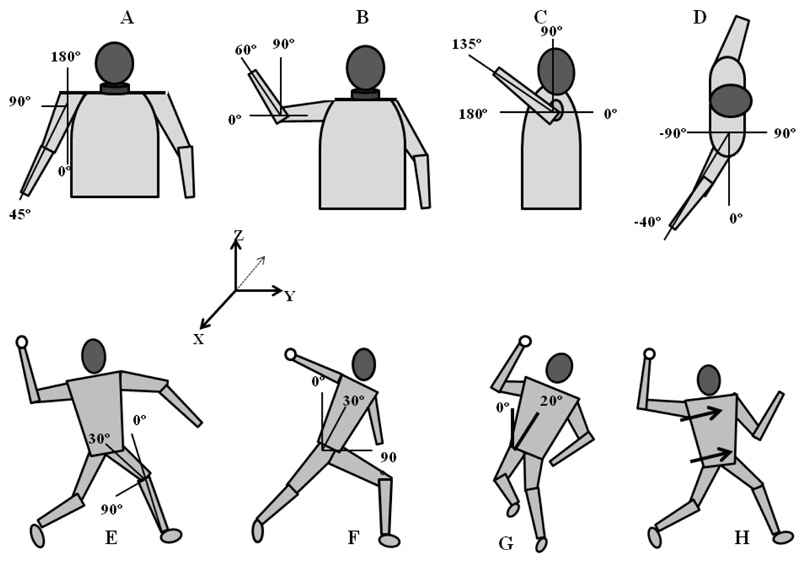
Lead Foot Contact Phase | Pre-fatigue | Fatigue | ||
---|---|---|---|---|
Mean | SD | Mean | SD | |
Stride length height (cm)* | 68.4 | 7.8 | 53.6 | 6.4 |
Shoulder abduction (º) | 103.5 | 12.3 | 97.5 | 11.2 |
Shoulder external rotation (º) | 99.1 | 17.7 | 94.1 | 16.9 |
Shoulder horizontal adduction (º) | 25.5 | 1.34 | 23.9 | 1.25 |
Knee flexion (º) | 130.2 | 20.3 | 128.5 | 19.6 |
Elbow flexion (º)* | 82.7 | 11.6 | 70.7 | 10.2 |
Arm Cocking Phase | ||||
Maximum shoulder external rotation (º/s) | 161.6 | 6.3 | 158.4 | 5.8 |
Maximum shoulder horizontal adduction (º/s)* | 155.7 | 7.3 | 140.5 | 9.3 |
Maximum elbow flexion (º/s) |
69.5 |
8.3 |
67.1 | 7.7 |
Maximum pelvis angular velocity (º/s) |
622.4 |
70.6 |
613.6 |
71.5 |
Maximum upper torso angular velocity (º/s) | 1224.5 | 111.3 | 1185.8 | 109.4 |
Arm Acceleration Phase | ||||
Shoulder abduction (º) | 107.3 | 8,1 | 101.4 | 7.8 |
Maximum elbow extension rotation (º/s)* | 2361.8 | 29.2 | 2021.4 | 24.3 |
Maximum shoulder internal rotation (º/s)* | 2617.3 | 21.2 | 2134.7 | 26.7 |
Ball Release Phase | ||||
Knee flexion (º) | 134.9 | 10.8 | 131.4 | 10.1 |
Forward trunk tilt (º) | 39.7 | 5.5 | 35.1 | 5.1 |
Lateral trunk tilt (º)* | 29.9 | 10.9 | 15.1 | 8.2 |
Angle elbow flexion (º) | 140.3 | 19.1 | 136.6 | 18.4 |
Shoulder horizontal adduction (º) | 56.5 | 5.8 | 54.5 | 5.4 |
Ball velocity (m·s−1) | 18.7 | 1.5 | 12.1 | 1. 4 |
Elbow flexion is the angle between the distal directions of the upper arm vector and the forearm vector. Pelvis angular velocity is calculated as the cross-product of a vector through the 2 hip markers and the first derivative of this vector. Similarly, the upper trunk angular velocity is calculated as the cross-product of a vector through the shoulder joint centers and the first derivative of this vector.
2.5. Data Analysis
For data analysis, the SPSS program version 21.0 for Windows was used. Descriptive statistics (mean ± SD) was performed. A paired sample t-test was applied to identify differences between pre-fatigue and post-fatigue conditions in terms of maximal ball throwing with the degree of confidence of 95%. The position-time data were filtered with a fourth-order Butterworth low-pass filter with a cutoff frequency of 13.5 Hz. The midpoint of the throwing wrist was defined as a midpoint between the medial and lateral wrist markers, while throwing shoulder and elbow joint centers were calculated using the previously described approaches [28, 29].
3. RESULTS
Table 1 shows the results of data analysis of 12 pitchers (mean, standard deviation, and significant differences) at the lead foot contact, arm cocking, arm acceleration, and ball release phases, descriptions of each indicator under fatigue and pre-fatigue conditions are presented.
Table 1 shows that there is a significant difference in the ball velocity under fatigue (18.7 m·s−1) and pre-fatigue (12.1 m·s−1) conditions with p = 0.042. There were significant differences in the stride length percentage height (p = 0.041) and elbow flexion (p = 0.046) on the velocity of ball pitching during the lead foot contact phase, in the maximum shoulder horizontal adduction (p = 0.041) on the ball pitching velocity in the arm cocking phase, in the maximum elbow extension angular velocity (p = 0.035), in the maximum shoulder internal rotation (p = 0.029) on the velocity of ball pitching during the arm acceleration phase, and in the lateral trunk tilt (p = 0.029) on the velocity of ball pitching during the ball releasing phase under fatigue condition.
4. DISCUSSION
The results of the analysis of this research focus on the kinematics of motion of the pitcher using 12 indicator parameters divided into four phases, including lead foot contact, arm cocking, arm acceleration, and ball release phases. The motion of a pitcher involved a coordinated movement from the lower and upper muscle groups to push the ball with the aim of creating the maximum velocity. The ball velocity reached 17.7 m·s−1 when the pitcher was in the pre-fatigue condition and 12.1 m·s−1 under the fatigue condition.
The result showed significant differences in the elbow flexion, maximum shoulder horizontal abduction, maximum elbow extension angular velocity, maximum shoulder internal rotation, and lateral trunk tilt under the fatigue condition. The main contribution of the rotation motion of the elbow extension angular velocity and internal shoulder rotation when pitching the ball is observed not only in baseball, but also in badminton during a smash [12], in tennis service [30], overhead water polo throwing [31], and overhead handball throwing [32-34]. The results of a study by Trigt, Schallig, & Graaff. (2018) showed that the lateral trunk tilt had a significant relationship with the ball velocity during the ball release. This result is consistent with those in several studies related to the role of trunk tilt as the major support of the sequence of the kinetics of pitching to produce the maximum ball velocity. An increase in the lateral trunk tilt angle, which is far from the hand during pitching, may improve ball velocity [17, 15, 13].
Changes in kinematics are the primary outcome of pitching-induced muscle fatigue. With an increase in the muscle fatigue, trunk flexion is altered during the arm cocking and acceleration phases, in addition, changes in shoulder and elbow joints are observed [35-36]. Wicke et al. (2013) observed that trunk kinematics changed; however, other kinematic and kinetic variables were unaffected. The authors noted that a more rigorous fatigue protocol may provide additional insight.
CONCLUSION
Cardiorespiratory fatigue causes changes in the kinetic harmonization of upper and lower body motion, which results in a decrease in the ball velocity. These results are similar to those in previous studies, which were conducted with the intervention of muscle fatigue during overhead baseball throwing, which caused a decrease in the performance. The main contribution of the elbow extension angular velocity and internal shoulder rotation when pitching the ball is observed not only in baseball, but also in badminton during a smash, in tennis service, and overhead handball throwing.
ETHICS APPROVAL AND CONSENT TO PARTICIPATE
The study was conducted in accordance with the recommendations of the ethics committee of the Universitas Pendidikan, Indonesia.
HUMAN AND ANIMAL RIGHTS
No Animals were used in this research. All human research procedures followed were in accordance with the ethical standards of the committee responsible for human experimentation (institutional and national), and with the Helsinki Declaration of 1975, as revised in 2013.
CONSENT FOR PUBLICATION
All participants were informed about the protocol and gave their written informed consent before participating in the study.
AVAILABILITY OF DATA AND MATERIALS
The datasets analyzed during the current study are available from the corresponding author [AR] on reasonable request.
FUNDING
None.
CONFLICT OF INTEREST
The authors declare no conflict of interest, financial or otherwise.
ACKNOWLEDGEMENTS
KEMENDIKBUD RI (grant-in-aid in Penelitian Dasar Unggulan Perguruan Tinggi (PDUPT) and Universitas Pendidikan Indonesia are acknowledged for supporting this research.