All published articles of this journal are available on ScienceDirect.
Limited Post-activation Potentiation Effects Provided by the Walking Lunge on Sprint Acceleration: A Preliminary Analysis
Abstract
Background:
Bilateral strength exercises may not provide a movement-specific stimulus to achieve post-activation potentiation (PAP) for sprinting. The walking lunge (WL) could provide this, due to its unilateral action similar to the running gait.
Objective:
To determine whether the WL potentiated 20-m sprint performance.
Method:
Nine strength-trained individuals (six men, three women) completed a five-repetition maximum (5RM) WL in one session, and two PAP sessions (control condition [CC] of 4 minutes rest and 5RM WL). Subjects were assessed in baseline 20-m (0-5, 0-10, 0-20 m intervals) sprints, and sprints ~15 s, 2, 4, 8, 12, and 16 min post-PAP intervention. Repeated measures ANOVA calculated significant changes in sprint times. The best potentiated time for each interval was compared to the baseline to individualize subject recovery times. Effect sizes (d) were derived for magnitude-based inference comparisons between the baseline and all sprints. Sprint potentiation and strength measured by the WL were also correlated (p < 0.05).
Results:
There were no significant interactions between the PAP conditions and any time point (p = 0.346-0.898). Magnitude based-inference showed a trivial 0.72% decrease in 0-5 m time 4 min after the 5RM WL. There was a moderate potentiation effect following the 5RM WL for the 0-5 m interval best time (d = 0.34). The correlation data did not suggest that greater strength in the WL aided sprint potentiation.
Conclusion:
The 5RM WL did not significantly potentiate sprint speed. The WL requires stability and control which could limit the applied load and resulting potentiation.
INTRODUCTION
Post-activation potentiation (PAP) is a phenomenon in which muscular performance characteristics are improved due to their history of contraction, which is shown by an increase in rate of force development [1, 2]. There are different mechanisms said to be responsible for the PAP effect. Firstly, there is the phosphorylation of myosin regulatory light chains that results from the prior muscular contraction, which makes the actin and myosin more sensitive to Ca2+ [1, 2]. A second factor relates to the increased recruitment of higher order motor units, which should theoretically result in an increase in fast-twitch muscle fiber contribution to a contraction [1]. Tillin and Bishop [1] have also discussed how changes in the muscle pennation angle (the angle formed by the fascicles and the inner aponeurosis) could influence PAP. Notwithstanding the contribution of each of these factors to PAP, the force a muscle is able to produce following prior contractile activity is a result of the net balance between fatigue and potentiation [3].
Complex training, which typically involves completing a strength exercise with a load equal to or above 85% of an individual’s one-repetition maximum (1RM), followed by a power-based exercise such as a jump [4], is often utilized in an attempt to encourage PAP in athletes. There are a number of elements that will influence the fatigue and potentiation that may result within a complex pair. These include: the athlete’s training experience and level of strength, with stronger athletes experiencing greater PAP effects and potentiating sooner following a conditioning activity [5-8]; rest period length within the complex pair, with the optimal time being highly individual [7, 9-12]; intensity of the conditioning activity performed, which relates to the loading of the exercise [11, 13, 14]; and biomechanical similarities between the preload or conditioning activity and subsequent power-based exercise [10, 15]. The consideration of biomechanical similarities between the preload or conditioning activity and power-based exercise takes on greater importance considering the more recent investigations into PAP and sprint performance.
There is contention within the literature as to whether sprint performance can be potentiated by a strength exercise. The strength exercises commonly used have tended to be bilateral, which may provide limitations as to biomechanical specificity [16, 17], although the preload intensity can be delivered. For example, parallel back squats performed with 30-70% 1RM were found to potentiate the 10-20 m and 30-40 m intervals of a 40-m sprint after 4 minutes (min) in strength-trained men [16], while loads of 85% [13] and 90% [18] of 1RM improved 40-m sprint time after 4 min in soccer and Division III football players, respectively. When recovery periods were individualized in professional rugby players, Bevan et al. [19] found 0-5 m and 0-10 m sprint times were potentiated by 3 back squats performed with 91% of 1RM. In opposition to these findings, 30-m sprint times did not improve 4 min after performing a 3RM back squat in well-trained male and female track and field athletes [20]. A 3RM back squat also did not lead to improvements in 0-5 m [10], 0-10 m [10, 18], or 0-30 m sprint times [18] in strength-trained collegiate American football or rugby players. Division I collegiate male track and field athletes did not experience potentiation in a 40-m sprint 1 min after performing 3 power cleans with 90% of 1RM [15]. Till and Cooke [21] asserted that a set of 5RM deadlifts did not potentiate a 0-10 m or 0-20 m sprint after 4, 5, or 6 min recovery in youth soccer players.
As noted, one of the potential limiting factors in these studies investigating PAP effects upon sprinting was that the strength exercises (i.e. squats, deadlifts, power cleans) required bilateral support. Sprinting is a cyclic action, where the individual will alternate between unilateral ground contact and flight [22]. One of the key issues for an athlete in transferring general strength to the sprint step is ensuring that the neuromuscular system can control the augmented strength specific to the maximal running task [23]. Indeed, this is why unilateral strength exercises such as lunges [24, 25] have been recommended for speed training. The direction of force application could also influence whether a strength exercise can potentiate maximal running, and in particular the acceleration phase (i.e. the 0-20 m interval) of sprinting. For example, only Bevan et al. [19] illustrated that 10-m sprint performance can be potentiated by a strength exercise. Numerous other studies have illustrated that speed over distances less than 20 m were not potentiated by a strength-based conditioning activity [10, 15, 16, 18, 20, 21]. Yetter and Moir [16] stated that an exercise such as a back squat may not provide a movement-specific stimulation to the muscles required for sprint acceleration due to the different mechanical demands of acceleration versus maximal velocity sprinting. As sprint acceleration places a greater emphasis on horizontal force as opposed to vertical force [26], a strength exercise that features a horizontal component such as a walking lunge (WL) [25, 27] may be better suited to providing a PAP effect. However, no research has investigated if the WL is an appropriate exercise to potentiate sprinting.
As a result, this preliminary study investigated whether the WL can invoke a PAP response for a 20-m sprint, which included the 0-5 m, 0-10 m, and 0-20 m intervals. A load equating to a five-repetition maximum (5RM) WL was utilized [13, 21, 23, 28]. The effects on 20-m sprint performance following the 5RM WL were compared to a control condition (CC) of 4 min rest. The individual timing of potentiation for the subjects for each strength exercise was also monitored from the immediate completion of the exercise to 16 min post [10, 11, 29], and the best potentiated sprint time was also analyzed to individualize the PAP response for each subject [19]. The relationship between absolute and relative strength as measured by the 5RM WL and sprint potentiation was also investigated. It was hypothesized that the WL would potentiate 20-m sprint performance, and this would occur within the time frame of 4-8 min for most subjects. Additionally, greater strength would be related greater PAP effects for sprint acceleration.
MATERIAL AND METHODS
Subjects
Nine strength-trained individuals (age = 23.56 ± 1.67 years; height = 1.72 ± 0.10 m; body mass = 71.67 ± 14.34 kilograms [kg]) were recruited for this study. The sample included six males (age = 23.83 ± 1.17 years; height = 1.77 ± 0.06 m; body mass = 79.30 ± 10.36 kg) and three females (age = 23.00 ± 2.65 years; height = 1.61 ± 0.06 m; body mass = 56.40 ± 5.44 kg). Previous PAP research has combined males and females within their analysis [6, 14, 17, 30, 31], and therefore this approach was considered appropriate for this study. Subjects were required to: be currently strength training (≥three hours per week); have a strength training history (≥two times per week) extending over the previous year; be familiar with the WL; and not have any medical conditions compromising participation in the study. G*Power software (v3.1.9.2, Universität Kiel, Germany) was used to confirm that the sample size of nine was sufficient for a repeated measures ANOVA, within-factors analysis, and ensured the data could be interpreted with a moderate effect level of 0.35 [32], and a power level of 0.80 when significance was set at 0.05 [33]. This sample size is also similar to previous PAP research [10, 20, 21, 29-31, 34, 35]. The methodology was approved by the institutional ethics committee. Subjects received an explanation of the study, which included the risks and benefits of participation, and written informed consent was obtained prior to testing.
Procedures
Three testing sessions were used for this study, with at least 48-72 hours separating each session. The study design is shown in Fig. (1), and testing was completed at the same time of day across the sessions for each subject, depending on their availabilities. All testing was conducted in a teaching gym at the university. The first session involved determination of the 5RM for the WL. The order of the two PAP testing sessions (CC and WL) was randomized amongst the sample. Subjects wore their own athletic trainers for all tests, and in the 24-hour period prior to testing, abstained from intensive exercise. No knee wraps, weightlifting belts, or other supportive garments were permitted during the WL. At the start of the first testing session, the subject’s age, height, and body mass were recorded. Height was measured barefoot using a portable stadiometer (seca, Hamburg, Germany). Body mass was recorded by electronic digital scales (Tanita Corporation, Tokyo, Japan). Each session featured the same dynamic warm-up, which consisted of 5 min of jogging at a self-selected pace on a treadmill, 10 min of dynamic stretching, and progressive speed runs (~60%, 70%, 80%, and 90% of perceived maximum) over 20 m. Following this, subjects either progressed into the WL strength testing, or completed the baseline 20-m sprints.
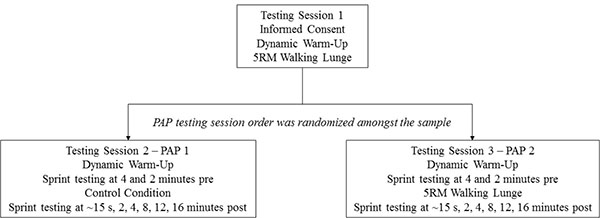
Strength Testing
The WL was performed with dumbbells according to established procedures [25, 27]. The 5RM referred to the loading for each leg, thus 10 repetitions in total were performed for the test. Standard dumbbells (American Barbell, San Diego, CA) were selected over a barbell due to greater ease of use, and the WL was performed across an unencumbered space on a gym floor. The warm-up for the WL was comprised of 10 repetitions for each leg at 50% of 1RM (as estimated by the subject) followed by 5 repetitions for each leg at 70% of 1RM, with 3 min recovery provided between sets. To complete the WL, the dumbbells were held in each hand with the arms by the subjects’ side. They then took a moderately large step forward with one leg (the subject self-selected their lead leg) and flexed at the hip and knee until the thigh was parallel to the ground, and the knee was positioned above the foot. The trail leg extended slightly at the hip and flexed at the knee, such that knee ended in a position approximately 5 centimeters (cm) above the floor. The trail leg was then flexed forward and completed the same action as the lead leg, which continued for the required number of repetitions. The torso remained erect throughout each repetition. A failed repetition, and thus test termination, occurred if the top of the thigh of the lead leg did not finish in a position parallel to the ground, the back knee contacted the ground, or the trunk flexed excessively forward [27]. The 5RM for the WL was generally determined within five attempts, and the last successful set of 10 repetitions was taken as the 5RM load (single dumbbell load x 2). The 5RM WL was also ratio scaled relative to body mass via the formula: relative load (kg·BM-1) = 5RM WL∙body mass-1.
PAP Testing
The same dynamic warm-up from the first session was performed at the start of each PAP testing session. Following the dynamic warm-up, each PAP session involved the performance of a single 20-m sprint at 4 min and 2 min before the strength set [10]. These two sprints were averaged and set the baseline for the sprints following the strength sets. 20-m sprint time was recorded by a timing lights system (Fusion Sports, Sumner Park, Australia). Gates were positioned at 0 m, 5 m, 10 m, and 20 m, at a height of 1.2 m and width of 2.5 m, to measure the 0-5 m [10, 22], 0-10 m [10, 18, 22], and 0-20 m intervals [21]. Subjects began the sprint from a standing start 50 cm behind the start line to trigger the first gate and were instructed to maximally sprint through all timing gates. Time for each interval was recorded to the nearest 0.001 s.
Two min after the second 20-m sprint, subjects completed one of two conditions: CC or WL (Fig. 1). The CC involved the subject having 4 min recovery in a seated position after the dynamic warm-up [21]. The other PAP testing session involved the subject performing the WL immediately after the dynamic warm-up. The loading selected for the WL (5 repetitions with 85% of 1RM) was based on previous research [13, 21, 23, 28]. Further, Yetter and Moir [16] stated that volume was more important than the actual load for eliciting a PAP response in sprinting. Taken together, this led to the adoption of a 5RM load in this study. As stated, subjects completed 5 lunges for each leg, which meant that 10 in total were performed. The WL was performed in the same manner as the strength test. Subjects initially completed 5 repetitions with approximately 70% for 1RM to prepare for the 85% load [16, 30], which was followed by 2 min recovery. The 5RM was then performed before the subjects progressed into the sprint tests.
After the PAP exercise, subjects completed a 20-m sprint at the following time points: ~15 s, and 2, 4, 8, 12, and 16 min [10, 11, 29]. Following established research, a single sprint was performed at each time interval [11, 29]. Using this approach, Turner et al. [11] found that 20-m sprint performance could be potentiated by alternate leg bounding, and alternate leg bounding while wearing a weighted vest, in strength-trained men. In contrast, Whelan et al. [29] found that resisted sprinting did not potentiate 10-m sprint performance in recreational male team sport athletes. Thus, the authors felt that if there was any potentiating effect to sprint performance, it would be due to the imposed load from the WL, and not the methods used to test sprint performance. Subjects were seated between each trial to reduce any effects of fatigue [10]. In addition to using the raw sprint time, the post-test sprints were compared to the baseline sprints according to the following formula: % Potentiation = Potentiated Variable (sprint performed at either ~15 s, 2, 4, 8, 12, and 16 min) ÷ Unpotentiated Variable (average baseline sprints) x 100 [6]. The percent potentiation values were used for the correlation analysis. A value equal to 100% meant no potentiation, less than 100% indicated PAP, and greater than 100% equated to post-activation depression [6].
Statistical Analysis
Statistical analyses were processed using the Statistics Package for Social Sciences (Version 24.0; IBM Corporation, New York, USA). Descriptive statistics (mean ± standard deviation [SD]) were calculated for each variable. A repeated measures ANOVA (2 x 7; within-subjects factors: condition [CC, BS] x time [baseline, ~15 s, 2, 4, 8, 12, 16 min]) was conducted [11]. Best potentiated sprint times for each participant, regardless of the time when it was achieved [19], were also investigated via a 2 (condition: CC, WL) x 2 (time: baseline, best) repeated measures ANOVA. Significance was set at p ≤ 0.05 for all analyses. Mauchly’s test of sphericity was checked, and the Greenhouse-Geisser correction was applied if sphericity was violated. If a significant F ratio was detected, post hoc tests were to be conducted using the Bonferroni adjustment procedure for multiple comparisons. Effect sizes (d) were derived for each of the PAP sprints relative to the baseline, where the difference between the means was divided by the pooled SD [36]. This magnitude-based inference analysis illustrated whether there were meaningful changes in sprint performance as a result of the imposed PAP condition [37]. A d less than 0.2 was considered a trivial effect; 0.2 to 0.6 a small effect; 0.6 to 1.2 a moderate effect; 1.2 to 2.0 a large effect; 2.0 to 4.0 a very large effect; and 4.0 and above an extremely large effect [32].
Spearman’s correlations (p ≤ 0.05) were calculated with respect to absolute and relative strength as measured by the 5RM WL, and the percentage changes in the time variables at ~15 s, 2, 4, 8, 12, and 16 min, and the best time. The strength of the correlation coefficient (ρ) was designated as per Hopkins [38]. A ρ value between 0 to 0.30, or 0 to -0.30, was considered small; 0.31 to 0.49, or -0.31 to -0.49, moderate; 0.50 to 0.69, or -0.50 to -0.69, large; 0.70 to 0.89, or -0.70 to -0.89, very large; and 0.90 to 1, or -0.90 to -1, near perfect for relationship prediction.
RESULTS
The mean 5RM WL for the sample was 43.34 ± 13.44 kg (range = 27.22-72.57 kg), which resulted in a mean relative strength measure of 0.61 ± 0.15 kg·BM-1 (range = 0.30-0.80 kg·BM-1). The PAP descriptive data for the 20-m sprint performances following the CC and WL, as well as the magnitude-based inference data, are shown in Table 1. There were no significant interactions between the PAP conditions and any of the time points for the 0-5 m (F6,42 = 0.788; p = 0.585), 0-10 m (F6,42 = 0.437; p = 0.850), or 0-20 m intervals (F6,42 = 0.369; p = 0.895). There were also no significant interactions between the PAP conditions and the best sprint times following the CC or WL for the 0-5 m (F1,8 = 0.130; p = 0.728), 0-10 m (F1,8 = 1.001; p = 0.346), or 0-20 m intervals (F1,8 = 0.018; p = 0.898).
CC | WL | |||
---|---|---|---|---|
Time (s) | d | Time | d | |
0-5 m | ||||
Baseline | 1.113 ± 0.082 | - | 1.109 ± 0.065 | - |
~15 s | 1.149 ± 0.104 | -0.38 | 1.117 ± 0.068 | -0.12 |
2 min | 1.130 ± 0.070 | -0.22 | 1.116 ± 0.056 | -0.12 |
4 min | 1.128 ± 0.080 | -0.19 | 1.101 ± 0.069 | 0.12 |
8 min | 1.131 ± 0.062 | -0.25 | 1.111 ± 0.053 | -0.03 |
12 min | 1.142 ± 0.083 | -0.35 | 1.113 ± 0.056 | -0.07 |
16 min | 1.132 ± 0.063 | -0.26 | 1.113 ± 0.057 | -0.07 |
Best | 1.091 ± 0.075 | 0.28 | 1.087 ± 0.063 | 0.34 |
0-10 m | ||||
Baseline | 1.904 ± 0.132 | - | 1.888 ± 0.129 | - |
~15 s | 1.966 ± 0.183 | -0.39 | 1.921 ± 0.134 | -0.25 |
2 min | 1.923 ± 0.123 | -0.15 | 1.902 ± 0.122 | -0.11 |
4 min | 1.915 ± 0.133 | -0.08 | 1.889 ± 0.128 | -0.01 |
8 min | 1.920 ± 0.128 | -0.12 | 1.900 ± 0.109 | -0.10 |
12 min | 1.938 ± 0.139 | -0.25 | 1.899 ± 0.113 | -0.09 |
16 min | 1.918 ± 0.113 | -0.11 | 1.893 ± 0.126 | -0.04 |
Best | 1.870 ± 0.126 | 0.26 | 1.865 ± 0.123 | 0.18 |
0-20 m | ||||
Baseline | 3.300 ± 0.278 | - | 3.284 ± 0.266 | - |
~15 s | 3.390 ± 0.359 | -0.28 | 3.363 ± 0.274 | -0.29 |
2 min | 3.318 ± 0.252 | -0.07 | 3.302 ± 0.254 | -0.07 |
4 min | 3.313 ± 0.273 | -0.05 | 3.272 ± 0.269 | 0.04 |
8 min | 3.333 ± 0.266 | -0.12 | 3.297 ± 0.243 | -0.05 |
12 min | 3.352 ± 0.276 | -0.19 | 3.303 ± 0.243 | -0.07 |
16 min | 3.347 ± 0.255 | -0.18 | 3.304 ± 0.247 | -0.08 |
Best | 3.253 ± 0.255 | 0.18 | 3.241 ± 0.254 | 0.17 |
The magnitude based-inference analysis showed that there was a trivial 0.72% decrease in 0-5 m sprint time 4 min after the 5RM WL. There were no other positive changes to sprint performance at any of the time points when considering the effect size data. With regards to the best times, there was a moderate 1.98% decrease in time following the 5RM WL for the 0-5 m interval, and trivial 1.22% and 1.31% decreases for the 0-10 m and 0-20 m intervals, respectively. There were small effects for the sprint potentiation of the best times following the CC in the 0-5 m and 0-10 m intervals (1.98% and 1.79% decrease, respectively), and a trivial effect for the 0-20 m interval (1.42% decrease).
The correlation data is displayed in Table 2. There were only three significant correlations out of 42 relationships. 5RM WL absolute strength had a positive correlation with percent sprint potentiation after 8 min for the 0-5 m interval, and after 16 min for the 0-20 m interval, and these relationships were large and very large, respectively. In both instances, this absolute strength data suggested that a greater WL load was associated with a greater percentage of baseline sprint performance, which is more indicative of post-activation depression. There was a very large negative correlation between 5RM WL relative strength and percent sprint potentiation for the 0-10 m interval at 12 min. This relationship demonstrated that greater relative strength was associated with a lower percentage of baseline sprint performance, which is indicative of PAP.
Absolute Strength | Relative Strength | |||
---|---|---|---|---|
ρ | p | ρ | p | |
0-5 m | ||||
~15 s | 0.197 | 0.612 | 0.200 | 0.606 |
2 min | 0.197 | 0.612 | -0.183 | 0.637 |
4 min | -0.137 | 0.726 | 0.067 | 0.865 |
8 min | 0.667 | 0.050* | 0.117 | 0.765 |
12 min | -0.111 | 0.793 | -0.571 | 0.139 |
16 min | 0.049 | 0.907 | -0.429 | 0.289 |
Best | 0.043 | 0.913 | -0.133 | 0.732 |
0-10 m | ||||
~15 s | 0.188 | 0.628 | 0.183 | 0.637 |
2 min | 0.120 | 0.759 | -0.350 | 0.356 |
4 min | 0.026 | 0.948 | -0.200 | 0.606 |
8 min | 0.567 | 0.112 | 0.033 | 0.932 |
12 min | -0.086 | 0.839 | -0.738 | 0.037* |
16 min | -0.037 | 0.931 | -0.524 | 0.183 |
Best | -0.060 | 0.878 | -0.400 | 0.286 |
0-20 m | ||||
~15 s | 0.308 | 0.420 | 0.033 | 0.932 |
2 min | 0.342 | 0.368 | -0.083 | 0.831 |
4 min | -0.060 | 0.878 | -0.217 | 0.576 |
8 min | 0.573 | 0.107 | 0.017 | 0.966 |
12 min | 0.136 | 0.748 | -0.643 | 0.086 |
16 min | 0.717 | 0.046* | 0.262 | 0.531 |
Best | 0.026 | 0.948 | -0.250 | 0.516 |
DISCUSSION
Strength and conditioning coaches can use complex training models such that strength exercises are performed prior to a power-based exercise such as sprinting in an attempt to invoke PAP. Previous research has shown that bilateral strength exercises can both potentiate [13, 16, 18, 19, 39] or have no effect [10, 18-21] on sprint performance. Potentially, bilateral exercises may not provide a movement-specific stimulus for sprint acceleration [16, 17]. Therefore, this study investigated the PAP effects of a more movement-specific strength exercise of a WL, as it incorporates both a vertical and horizontal force component during exercise execution [25, 27]. The results of this study indicated that the WL was not able to significantly potentiate 0-5 m, 0-10 m, or 0-20 m sprint performance in strength-trained males and females. The magnitude-based inference analysis indicated some small positive responses to the WL, but any positive changes were generally no different to a CC of 4 min rest. As will be discussed, these results suggest that the WL may not be an appropriate exercise to use for sprint potentiation.
When investigating whether a strength exercise could potentiate a sprint start, Cuenca-Fernandez et al. [17] commented that enhancement of general muscle performance was not enough; rather, a specific stimulus was required, especially as it relates to the control of the essential force vectors in the action. In conjunction with the suggestion by Yetter and Moir [16] that bilateral strength exercises may not provide preload specificity for sprint acceleration, this contributed to the analysis of the WL in this study. The WL incorporates both a vertical and horizontal force component within an action that is relatively similar to the running gait [24, 25], which theoretically could provide a potentiating stimulus to sprinting. Despite this, when considering the time course of PAP response from ~15 s to 16 min post intervention, the results indicated that the 5RM WL was not able to significantly potentiate any of the analyzed intervals in the 20-m sprint, which was counter to the studies’ hypothesis.
Magnitudes of difference were also considered when analyzing the 5RM WL data, as Buchheit [37] noted that this provides the most practical information for a coach or practitioner. For the WL, there was a trivial effect for sprint potentiation over the 0-5 m interval at the 4 min time point, but no positive effects for the 0-10 m or 0-20 m intervals. PAP effects for sprinting have been documented within the 4 min time frame [6, 10, 11, 13, 16, 19, 39]. Nonetheless, the load from the 5RM WL may not have been sufficient to induce a more meaningful change to sprint acceleration performance. Other studies investigating PAP responses for sprinting have also shown small percentage changes when utilizing relatively lighters loads during a preload exercise. For example, Till and Cooke [21] documented a 0.62% change in 0-20 sprint time when utilizing a 5RM deadlift as a preload exercise in youth soccer players. Rahimi [13] found a 1.09% decrease in 0-36.6 m sprint time following a low load back squat with 60% of 1RM in elite soccer players, while McBride et al. [18] noted a 0.97% decrease in 0-10 m sprint time in collegiate football players after a loaded countermovement jump with 30% of 1RM back squat. In each instance, including for the 5RM WL used in this study, the imposed load may not have been sufficient to induce a more meaningful sprint PAP response, given the importance of intensity of the conditioning activity for producing potentiation [11, 13, 14]. The data analyzed relative to the optimal recovery of each individual also supported this finding.
Nibali et al. [12] noted that the interpretation of mean data was not always appropriate for determining the time course of a PAP response, and it is important to ascertain the individual response for each athlete. This is because of the wide inter-individual variation that can occur with PAP responses to certain strength exercises [12, 19-21]. Thus, similar to Bevan et al. [19], the current study analyzed the best potentiated time, which essentially meant recovery time was individualized for each subject. The results indicated that there were no significant changes in the best potentiated sprint performance relative to the baseline for any sprint interval following either PAP condition. Sprint acceleration requires great force be produced to overcome the inertia of an individual’s body mass [22], with a particular importance for horizontal force production [26]. The force generated during the WL may not have been sufficient to potentiate 20-m sprint performance. Indeed, although there was moderate decrease in the best potentiated 0-5 m time, it did not differ to that for the CC. This was also true for the 0-10 m and 0-20 m intervals. The WL involves a greater need for stability and control, which is part of the reason why it is recommended for speed training [24, 25]. However, it could limit the actual load that can be applied, which may influence any resulting PAP effects. When attempting to potentiate sprint performance via the use of a resistance exercise, practitioners may be better served selecting an exercise where a high external load can be imposed.
This is reflected in the correlation data, where there were only three out of 42 relationships between sprint potentiation and absolute and relative strength were significant. Furthermore, only one significant relationship suggested that greater strength related to sprint potentiation. There was a very large relationship between relative strength, which is arguably more important within this study due to the combination of male and female subjects [40], and sprint potentiation in the 0-10 m interval 12 min post the 5RM WL. Several studies have acknowledged that stronger individuals are more likely to experience greater PAP in power-based exercises following a strength intervention [5-8]. This has been related to the fact that stronger individuals display elevated myosin light chain phosphorylation, and tend to have stronger and larger type II muscle fibers which exhibit greater neural excitation in response to strength training exercises [7]. However, due to the stability and control needs in the WL [24, 25], this may restrict the influence that lower-body strength has on this exercise being utilized as a preload PAP activity.
Although this study was preliminary and exploratory in nature, there are still limitations that should be acknowledged. The sample size was small (n = 9), although previous PAP research has used similar subject numbers [10, 20, 21, 29-31, 34, 35]. Nevertheless, a larger sample would increase the statistical power within the study. Although the subjects were strength-trained, they were not high-level athletes. An elite athlete may respond differently to a PAP intervention featuring a unilateral exercise such as a WL. A load equivalent to 85% of 1RM was adopted for the WL. Even though this was based on previous PAP research that has utilized this load [13, 21, 23, 28], there is also research that has found potentiation with heavier loads of approximately 90% of 1RM [18, 19, 39]. Forthcoming studies could analyze whether using a heavier load for the WL can potentiate sprint acceleration. However, performing the WL with a heavy load may be limited by the balance and stability required within the exercise [24]. As a result, other single-leg exercises where a greater load could be imposed, such as the Bulgarian split-squat or unilateral leg press, could be examined in future sprint PAP research. Practitioners and coaches could also experiment with using these exercises in practice to invoke the PAP effect for sprint performance. As an exercise such as the back squat may be limited by biomechanical dissimilarities to sprinting, while the WL does not appear to allow for an appropriate resistance, these unilateral exercises could prove beneficial. The Bulgarian split-squat or unilateral leg press could allow for a greater load to be imposed, will also providing some biomechanical similarity to sprinting due to the use of unilateral actions.
CONCLUSION
In conclusion, this study found that the 5RM WL did not significantly potentiate 20-m sprint performance in strength-trained men and women. Although there were some potential benefits found when utilizing magnitude-based inference analysis for 0-5 m time 4 min post the 5RM WL, and for best potentiated sprint times when individualizing recovery periods, any performance changes were not different to a CC of 4 min rest. Furthermore, absolute and relative strength as measured by the 5RM WL generally did not relate to any resulting sprint potentiation across any of the measured intervals (0-5 m, 0-10 m, and 0-20 m). The movement demands of the WL, where unilateral stability and control are stressed, may limit the load that can be imposed on the individual. As intensity of a preload exercise is important for invoking a PAP response, this could have reduced the effects of the 5RM WL on potentiating sprint performance. Within the context of the study limitations, the results from this research suggest that coaches should consider the use of other strength exercises instead of the WL within complex pairs designed to potentiate sprinting.
CONFLICT OF INTEREST
The authors declare no conflict of interest. This study received no external funding.
ACKNOWLEDGEMENTS
Robert G. Lockie was involved in study design, data collection, data interpretation, and manuscript writing. Adrina Lazar, Fabrice G. Risso, Dominic V. Giuliano, Tricia M. Liu, Alyssa A. Stage, Samantha A. Birmingham-Babauta, John J. Stokes, DeShaun L. Davis, Matthew R. Moreno, and Ashley J. Orjalo were involved in data collection, data interpretation, and manuscript editing. Thanks to Ibett Torne, Megan Beiley, and Jillian Hurley for assisting with data collection. We would finally like to acknowledge our subjects for their contribution to this study.