All published articles of this journal are available on ScienceDirect.
Effects of Low-Frequency Vibration on Physiological Recovery from Exhaustive Exercise
Abstract
Objective:
This study examined the effects of low-frequency vibration on physiological recovery from exhaustive exercise.
Methods:
Twelve college males were recruited in this randomized crossover-designed study, and were asked to perform one of three treatments following a graded cycling exercise test: nonvibration (0 Hz, 0 mm, CON), high-amplitude vibration (8 Hz, 8 mm, HVT), or low-amplitude vibration (8 Hz, 2 mm, LVT). After the 10-min treatment, participants were asked to rest in a supine position for a 1-h recovery. The oxygen uptake, heart rate (HR), and blood lactate concentration (La) were measured during the trials.
Results:
The oxygen uptake during HVT were significantly higher than those in the CON and LVT (p < 0.05, effect size = 1.52−1.63). The La immediately following HVT was significantly lower than that following CON (HVT vs. CON = 11.52 ± 1.85 vs. 12.95 ± 1.78 mmol•L-1, p < 0.05, effect size = 1.94). Additionally, the Las following HVT and LVT at the post 30-min were significantly lower than that following the CON (HVT vs. LVT vs. CON = 4.72 ± 0.97 vs. 4.58 ± 1.06 vs. 5.98 ± 1.49 mmol•L-1, p < 0.05). No significant differences were found on the HRs, or on the time and frequency domain indices of HR variability among treatments during the recovery period.
Conclusion:
These results indicated that vibration with low frequency (8 Hz) can facilitate the removal of metabolic by-products after exhaustive exercise, but it has little effect on the autonomic nervous modulation of HR recovery.
INTRODUCTION
Achieving an appropriate balance between training or competition stress and recovery plays an essential role in optimizing athletes’ performances. Numerous recovery modalities (e.g., massage, light activities, and cryotherapy) have been proposed to attain this balance by increasing blood flow to and from the damaged muscle, removing waste by-products, reducing muscle soreness, and stimulating the muscle receptors to reduce muscular tension following high-intensity exercise [1, 2]. Vibration is a mechanical oscillation whereby energy is transferred from the vibration device to human body. Vibration exercise, in which intensity is administered by frequency and amplitude (peak-to-peak displacement) of vibration platform, can increase muscular activity [3] and muscle deoxygenation [4, 5], and result in an increase in the oxygen uptake () and energy expenditure, which is similar to the effects of light exercise [6, 7]. Previous studies have also reported that the vibration exercise can increase peripheral circulation [8], muscle temperature [9], and skin [10, 11] and leg blood flow [12]. Therefore, the vibration exercise may act as a mechanical massage to facilitate physiological recovery from strenuous exercise.
Several studies have shown that the frequency of vibrations higher than 20 Hz could improve both delayed onset muscular soreness (DOMS) and physiological recovery after exhaustive exercise. When performing vibration exercise, it is not possible to apply a high frequency with high amplitude. Normally, vibration exercise with higher frequency is performed with low amplitude. After an exercise which triggered DOMS, a local [13] or whole-body [14, 15] vibrational stimulation for consecutive 3−4 days has facilitated the improvements in DOMS and range of motion, thereby accelerating the recovery of muscles [16]. In other studies, participants have been asked to sit and place their feet on a vibration platform immediately after exhaustive exercise to perform a vibration exercise (25 Hz, 4 mm). This treatment accelerated the decline of the heart rate (HR) during recovery [17], increased peripheral vasodilation, and improved the following time to exhaustion performance [12]. One study [18] found that vibration exercise with a high frequency and low amplitude (30 Hz & 40 Hz, 2.4 & 4.4 mm) can also improve muscle oxygenation after exhaustive exercise. Its effect of improving the subsequent Wingate test performance was similar to active recovery. However, it was much less effective with regard to blood lactate removal 30 minutes after exercise compared with active recovery. Nevertheless, not all studies support the assumption that vibration exercise facilitates physiological recovery after exercise. For example, Cheng et al. [19] found that vibration exercise with 20 Hz and 36 Hz frequencies, and 0.8 mm improved neither the recovery of HR and , nor the removal of blood lactate after exhaustive exercise. Carrasco et al. [20] similarly found that vibration exercise with 20 Hz frequency and 4 mm improved neither the blood lactate removal and muscle contractile properties after exhaustive exercise, nor the time to exhaustion of the subsequent high-intensity exercise. Furthermore, Rittweger et al. [21] suggested that a vibration frequency below approximately 20 Hz may induce muscular relaxation according to experiences from their laboratory. However, to the present authors’ knowledge, no study has yet explored the effect of low-frequency with high amplitude vibration followed by an intense exercise. One study about low frequency and high amplitude indicated that the
reaction triggered by vibration exercise (3−6 Hz, 6 mm) was similar to the effects of light activity [22]. Therefore, we hypothesized that exercise with a low-frequency vibration and high amplitude may accelerate physiological recovery after exhaustive exercise.
The autonomic nervous system adjusts the HR through a balance of sympathetic and parasympathetic pathways. Time and frequency domain analyses of HR variability (HRV) based on RR intervals have been considered credible and noninvasive tools that can be used to assess the regulatory effects of the autonomic nervous system on the sinus node of the heart [23, 24]. The HR returns to a resting state after exercise mainly because of parasympathetic activation or sympathetic withdrawal [25-27]. Previous studies have determined that vibration exercise in either a standing (20 Hz and 36 Hz) [19] or sitting (25 Hz) [17] pose did not change the indices of HRV during the recovery period following exhaustive exercise. However, the simulation of vehicle vibration with a low-frequency (6 Hz) vibration was found to affect the frequency domain indices of HRV [28]; in short, it increased sympathetic activity and reduced parasympathetic activation. Therefore, another purpose of this study was to investigate whether vibration exercise with low frequency and high amplitude influences the regulatory effects of the autonomic nervous system on HR during the recovery period following exhaustive exercise.
MATERIALS AND METHODS
Participants
Twelve healthy male college students (age = 20 ± 2 years; height = 1.77 ± 0.06 m; body mass = 72 ± 7 kg) were voluntarily recruited for this study. All participants completed a medical history and health questionnaire, and provided written informed consent before participating in the experimental procedures. The participants were asked to abstain from exercise for 24 hours, and to fast at least 4 hours prior to visiting the laboratory, to reduce the interference effects of the exercise and food. The study was approved by the Taipei Medical University-Joint Institutional Review Board.
Procedures
A randomized, crossover trial design was used in this study to eliminate any order effects. The testing protocol required four visits to the laboratory over a 3-week period. During the first visit, participants performed the graded exercise test (GXT) on an electrically braked cycle ergometer (Cyclus 2, h/p/cosmos, Germany), which served only as a familiarization trial and was not included in the data analyses. During each participant’s first visit to the laboratory, the cycling ergometer seat and handlebars were adjusted for comfort; these same settings were then restored for each consecutive exercise trial. Over the following three visits (separated by at least 48 hours), a nonvibration (CON, 0 Hz, 0 mm), low-amplitude vibration (LVT, 8 Hz, 2 mm), or high-amplitude vibration (HVT, 8 Hz, 8 mm) treatment was administered to the participants following their GXTs Fig. (1). The amplitude of vibration treatment in this study was defined as the peak-to-peak displacement of periodic oscillation. The participants completed all of the trials during the same period (± 2 h) of testing days to eliminate any effect from circadian variation.

Before the GXTs, participants were asked to rest in a supine position for at least 20 min for the baseline measurements, and then performed a standardized warm-up (3 min, 50 watts, 60–80 rev·min-1) on the cycling ergometer. Similar to a previous study [29], the participants performed a GXT in which the exercise load was gradually increased. The GXT began at an initial workload of 150 watts and a pedaling rate of 60 rev·min-1 for 2 minutes, followed by increasing increments of 30 watts every 2 minutes until exhaustion. Strong verbal encouragement was provided throughout the trial. Exhaustion was defined by attainment of at least three criteria [30]: (1) a respiratory exchange ratio > 1.2; (2) HR > 90% of age-predicted maximum; (3) a plateau of defined as no change (< 150 mL·min-1) in
from the previous test stage; or (4) rating of perceived exertion > 17 on the Borg’s 6–20 scale.
Pulmonary gas exchanges were measured breath-by-breath throughout the GXTs by having the participants wear a face mask (7400 Vmask series, Hans Rudolph, Kansas City, MO, USA) attached to a portable gas analysis system (Cortex Metamax 3B; Cortex Biophysik, Leipzig, Germany). Before the trial, the system was calibrated according to the manufacturer’s guidelines against known concentrations of cylinder gases (15% oxygen, 5% carbon dioxide) and a 3-L calibration syringe (5530 series, Hans Rudolph, Kansas City, MO, USA). HRs was monitored using a telemetry system with a wireless chest strap (Polar S810i; Polar Electro, Inc., Oy, Kempele, Finland) and continuously measured through a link to the Cortex gas analysis system during the exercise test. The greatest and HR value (averaged every 30 s) measured during the GXT was respectively recorded as the
max and HRmax value.
Immediately following the GXT, the participants were seated on a chair with their feet placed on a vertical vibration device (AV-001A, Body Green Technology Co. Ltd., Taiwan) for a 10-min treatment of CON, LVT, or HVT. After the vibration treatments, the participants were asked to rest in a supine position for 60 min to monitor the recovery phase. The average of and HR was analyzed during the last 5 min of the baseline phase (pretest), treatment, and at the 30-min (25–30 min after treatment) and 60-min (55–60 min after treatment) mark during the recovery phase. The capillary blood samples were obtained by a finger puncture at the pretest, immediately after GXT and treatment, and at the 30- and 60-minute mark during the recovery phase. The first blood samples were discarded, and the second blood samples (approximately 5 µL) were used to analyze the blood lactate concentrations (Las) using a lactate chemistry analyzer (Lactate Pro, Arkray, Inc., Japan). The Lactate Pro analyzer was calibrated using check or calibration strips provided by the manufacturer 30 min before testing the capillary blood samples, thus ensuring the correct operation and precision of the analyzer.
The HR data were transferred via Polar infrared rays (Polar IR Interface; Polar Electro Inc.) to a personal computer; the 5 min data, including from the pretest, post-30 min, and post-60 min, were selected for HRV analysis. Before processing, the HR signals were automatically corrected by the Polar ProTrainer 5.0 package software for ectopic and missed beats, and then the modified HR data were transformed into ASCII files. The time domain and frequency domain analyses of RR intervals were performed by HRV analysis software (Kubios HRV version 2.0; University of Kuoplo, Finland). In the time domain analysis, the RR mean, standard deviation of all normal RR intervals (SDNN), and the square root of the mean squared successive differences between adjacent RR intervals (RMSSD), were evaluated from the electrocardiogram data. To analyze the frequency domain indices, the HR waveform was resampled at 4.0 Hz, and the spectral power was derived through a 1024-point linear fast Fourier transformation with a Hamming window. The power spectrum was then analyzed for LF (0.05–0.15 Hz) and HF (0.15–0.40 Hz) powers. Because the raw values of LF and HF did not follow a normal distribution, a natural log (ln) transformation was applied (lnLF and lnHF) [31]. Sympathovagal balance was estimated by the ration of lnLF: lnHF. Finally, the coefficient of component variance (CCV) of the LF- and HF-power was calculated using the following formula [32]: CCVA (%) = 100 × (power of component A)1/2/(RR mean).
Statistical Analysis
SPSS for Windows software (version 17.0, SPSS, Inc., Chicago, IL, USA) was used to conduct the statistical analysis. All data were expressed as mean ± standard deviation (SD). A two-way (3 treatments × 5 time points) within-participants repeated measures ANOVA was used to investigate differences in the La, , HR, and HRV (3 treatments × 3 time points) indices. If a significant interaction was found, a subsequent one-way ANOVA with Bonferroni post hoc comparison was performed to examine the differences over time and between treatments. The effect size (ES) and 95% confidence intervals (95% CI) were also calculated to evaluate differences between treatments. The ES was calculated by dividing the difference between mean values of the treatments by the pooled SD [33]; the ES values of < 0.5, 0.5−0.79 and ≥ 0.8 were considered to represent small, moderate, and large effects, respectively [33]. Statistical significance was denoted by a p value of ≤ 0.05.
RESULTS
There were no significant differences in the workload at max (HVT vs. LVT vs. CON = 263 ± 29 vs. 263 ± 32 vs. 265 ± 28 W, F = 0.234, p > 0.05) and total caloric expenditure (HVT vs. LVT vs. CON = 127 ± 29 vs. 129 ± 32 vs. 132 ± 30 kcal, F = 2.292, p > 0.05) during GXT among the treatments. The results of the
and HR data for the different treatments are represented in Table 1. Significant interaction effects were found for
(F = 2.191, p < 0.05). No significant differences in
max were found among the treatments (HVT vs. LVT vs. CON = 49 ± 6 vs. 50 ± 6 vs. 50 ± 5 mL·kg-1·min-1, p > 0.05), as well as the
during the pretest. However, the
during the HVT treatment was significantly higher than those in the CON (p < 0.05, ES = 1.52, 95% CI = 0.47−2.03) and LVT (p < 0.05, ES = 1.63, 95% CI = 0.57−2.11) treatments. There were also no significant differences in the
at any of the 30-min or 60-min post-treatment marks. No significant interaction effects were determined for HR (F = 1.624, p > 0.05), but a significant main effect was found for time (F = 1341.7, p < 0.05).

Pre-test | GXT | Treatment | Post-30 min | Post-60 min | |
---|---|---|---|---|---|
HR (bpm) | |||||
HVT | 64 ± 9 | 186 ± 7 | 113 ± 7 | 80 ± 9 | 75 ± 8 |
LVT | 61 ± 5 | 186 ± 8 | 113 ± 7 | 83 ± 8 | 75 ± 7 |
CON | 62 ± 8 | 187 ± 8 | 109 ± 16 | 84 ± 11 | 77 ± 9 |
![]() |
|||||
HVT | 4.5 ± 1.3 | 49.1 ± 5.9 | 10.0 ± 1.9*† | 5.5 ± 1.2 | 5.1 ± 1.1 |
LVT | 4.4 ± 0.7 | 49.7 ± 6.3 | 8.2 ± 0.8 | 5.5 ± 0.7 | 4.9 ± 0.8 |
CON | 4.6 ± 0.8 | 50.4 ± 4.9 | 8.3 ± 0.9 | 5.2 ± 0.7 | 4.9 ± 0.7 |
Moreover, significant interaction effects were found for Las (F = 2.690, p < 0.05). Fig. (2) illustrates that the La after HVT treatment was significantly lower than that following CON treatment (HVT vs. CON = 11.52 ± 1.85 vs. 12.95 ± 1.78 mmol·L-1, p < 0.05, ES = 1.94, 95% CI = 0.94−2.99). Additionally, the Las in the HVT (4.72 ± 0.97 mmol·L-1, p < 0.05, ES = 1.69, 95% CI = 0.85−2.24) and LVT (4.58 ± 1.06 mmol·L-1, p < 0.05, ES = 2.33, 95% CI = 1.49−2.93) treatments at post-30 min were significantly lower than that in the CON treatment (5.98 ± 1.49 mmol·L-1). No significant differences were found in Las at pre-test, after GXT, and at 60 min post-treatment in the CON, HVT, or LVT.
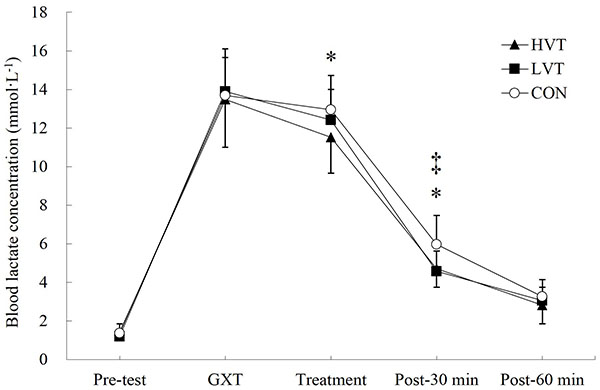
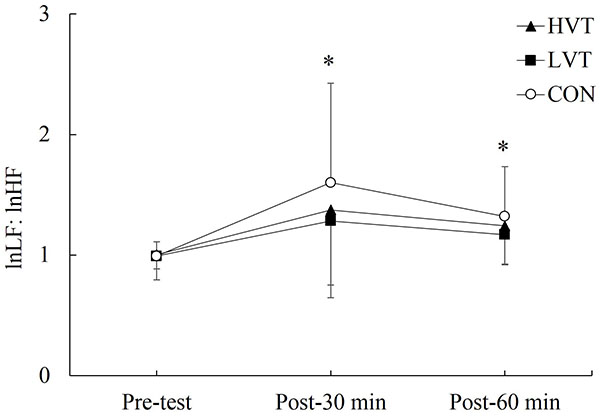
Table (2) displays the effect of various vibration treatments on HRV indices. No significant interaction effects were determined for the time domain indices of HRV (p > 0.05), but a significant effect was found for time (p < 0.05). Although no significant differences were found on the time domain indices of HRV at each time point among treatments (p > 0.05), the mean RR, SDNN, and RMSSD at post-30 min in each treatment were significantly lower than those during the pretest (p < 0.05). The mean RR at post-60 min among all of the treatments, and the RMSSD at post-60 min in the LVT and CON was significantly lower than those during the pretest. In addition, the time domain indices of HRV at post-60 min in the three treatments were significantly higher than those at post-30 min, except the RMSSD in the LVT. Furthermore, significant interaction effects were determined for the frequency domain indices of HRV (p < 0.05), except the CCVHF. No significant differences were found at any of the time points among the treatments for the frequency domain indices of HRV. However, the lnLF, lnHF, and CCVHF at post-30 min, and the lnHF and CCVHF at post-60 min in the CON treatment were significantly different from those during the pretest Table (2). As Fig. (3) depicts, the lnLF: lnHF at the 30-min (p < 0.05, ES = 3.34, 95% CI = 2.87−3.41) and 60-min (p < 0.05, ES = 2.91, 95% CI = 2.67−2.97) marks in the CON treatment were significantly higher than that during the pretest, whereas no significant differences between the pretest and recovery phase were found in the HVT and LVT treatments.
Pre-test | Post-30 min | Post-60 min | |||||||
---|---|---|---|---|---|---|---|---|---|
HVT | LVT | CON | HVT | LVT | CON | HVT | LVT | CON | |
Mean RR (ms) | 956 ± 135 | 993 ± 97 | 998 ± 131 | 761 ± 91a | 735 ± 74a | 732 ± 103a | 818 ± 97a, b | 809 ± 81a, b | 795 ± 107a, b |
SDNN (ms) | 69 ± 22 | 76 ± 39 | 87 ± 31 | 42 ± 17a | 40 ± 17a | 34 ± 19a | 60 ± 18b | 64 ± 23b | 64 ± 35b |
RMSSD (ms) | 58 ± 33 | 61 ± 32 | 74 ± 40 | 23 ± 17a | 21 ± 14a | 17 ± 16a | 31 ± 18b | 36 ± 21a | 28 ± 20a, b |
lnLF (ms2) | 6.65 ± 0.65 | 6.47 ± 1.13 | 7.24 ± 0.96 | 5.90 ± 0.97 | 5.60 ± 1.17 | 5.36 ± 1.32a | 6.62 ± 0.66b | 6.69 ± 1.10b | 6.47 ± 0.98b |
lnHF (ms2) | 6.85 ± 1.30 | 6.63 ± 1.49 | 7.35 ± 1.09 | 4.94 ± 1.81a | 5.01 ± 1.70 | 4.07 ± 1.86a | 5.67 ± 1.53b | 5.97 ± 1.56 | 5.32 ± 1.67a, b |
CCVLF (%) | 3.11 ± 1.11 | 2.86 ± 1.20 | 4.20 ± 2.14 | 2.72 ± 1.18 | 2.56 ± 1.35 | 2.33 ± 1.35 | 3.47 ± 0.84 | 3.90 ± 1.83 | 3.48 ± 1.39 |
CCVHF (%) | 3.71 ± 1.89 | 3.35 ± 1.91 | 4.44 ± 2.02 | 2.01 ± 1.32 | 2.12 ± 1.35 | 1.42 ± 1.13a | 2.51 ± 1.42 | 3.03 ± 1.95 | 2.28 ± 1.58a, b |
DISCUSSION
The primary aim of this study was to examine the effects of a low frequency and high amplitude (8 Hz, 8 mm) vibration exercise on the recovery from exhaustive cycling exercise. The major finding of this study was that the low frequency vibration exercise could speed up the blood lactate recovery following strenuous exercise. Although low frequency vibration exercise could not accelerate the decline in HR and , such a recovery modality might improve the regulation of the autonomic nervous system on the heart through increased parasympathetic reactivation.
Overall, the energy consumption increased with the increasing intensity of the vibration exercise. For instance, under the same frequency of vibration, increased at higher amplitudes [6]. When the vibration exercise (26 Hz) with different amplitudes (2.5 mm & 7.5 mm) was performed in a squatting position, the
reached 0.31 and 0.53 L·min-1, respectively [6]. Other previous studies on vibration exercise with low-frequency (3−8 Hz) [22, 34] performed in a seated position found that the energy consumption produced by vibration exercise (approximately 0.24−0.27 L·min-1) was similar to that of light activity. In the present study, the
produced by low-frequency vibration exercise performed in any treatment (0.59 L·min-1 for LVT, 0.71 L·min-1 for HVT) was higher than in the previous studies because of the diverse experimental situations; specifically, the treatments in this study were performed after exhaustive exercise, resulting in higher energy consumption. Nevertheless, the results of this study also revealed that compared with controlling treatment, the
of the HVT significantly increased by 0.12 L·min-1 during recovery, whereas the energy consumption of the LVT was similar to the CON. Another prior study [19] also revealed that vibration exercise with low frequency and low amplitude (20 Hz, 0.8 mm) did not affect the
during recovery after exhaustive exercise, that is, the excess postexercise oxygen consumption caused by exhaustive exercise might obscure the impact of vibration exercise with a lower intensity on the
during recovery period. Nevertheless, vibration exercise with low frequency and high amplitude has been demonstrated to increase energy consumption during recovery following exercise, as well as passively stimulate the body.
This study is the first to discover that vibration exercise with low-frequency facilitates the removal of blood lactate following exhaustive exercise; however, it did not improve the or HR during the recovery period. Although several studies [1, 35] discuss the validity of the La as a parameter to determine the postexercise muscle recovery, this biochemical marker has been widely used for such purpose [36-38]. Researchers have also determined that a vibration frequency over 20 Hz (25−30 Hz, 2−6 mm) facilitates the reduction of arterial stiffness [39], the acceleration of blood velocity [8], and the increase of both muscle temperature [9] and blood flow [10-12]. However, other studies have suggested that a vibration frequency over 20 Hz (20−40 Hz, 0.8−4.4 mm) cannot improve the removal of blood lactate during the recovery period after exhaustive exercise [18-20]. For example, Manimmanakorn et al. [18] argued that after six sets of 30-s Wingate sprints, vibration exercise (30 & 40 Hz, 2.4 & 4.4 mm) increased muscle oxygenation compared with active and passive recovery conditions, thus generating a vasodilation effect; however, they also revealed that the La after 30 min of vibration exercise was significantly higher than that of active recovery treatment. Furthermore, some studies have indicated that a vibration frequency of less than 20 Hz (10−16 Hz, 4−5 mm) enhanced muscle oxygenation [4, 5] and increased blood velocity and skin temperature [40]. Therefore, high-intensity vibration (e.g., frequency > 20 Hz) does not facilitate the removal of blood lactate after exercise, but low-intensity vibration (e.g., < 20 Hz) may facilitate the recovery of muscles after exercise. Regardless, accelerating the removal of blood lactates with a low-frequency vibration exercise following exercise still requires further exploration.
During the recovery period following exercise, HR has been shown to gradually decline because of parasympathetic activation or sympathetic withdrawal [25-27]. Previous studies have found that the stimulation from vibration exercise changes modulation of the autonomic nervous system, and low-frequency vibration exercise (6 Hz) increases the sympathetic activity and reduces parasympathetic activation [28]. Therefore, the application of low-frequency vibration may disturb the regulation of the autonomic nervous system on HR recovery from exercise. The results of this study demonstrated that vibration exercise with low frequency did not affect cardiac autonomic response during the recovery period after exhaustive exercise, which is consistent with previous findings [17, 19]. However, an examination of the time variables (Table 2 and Fig. 3) suggested that low-frequency vibration exercise might increase the parasympathetic reactivation. Cheng et al. [19] found that the regulation of the autonomic nervous system during the recovery period after exercise showed no significant differences among different frequencies (20 & 36 Hz). However, a 20-Hz whole-body vibration performed in a standing position during recovery after exercise might improve the parasympathetic activation following exhaustive exercise [19]. In a study by Sanudo et al. [17], participants were seated with both feet placed on a platform for a 25-Hz vibration exercise. Their total HRV power increased after exhaustive exercise, but the vibration did not affect LF or HF components of HRV. Nevertheless, vibration exercise with either high- or low-frequency had little impact on the activity of the autonomic nervous system during the recovery period after exercise because the stimulation of exhaustive exercise for the autonomic nervous system [27, 41, 42] was much higher than that of the lower intensity vibration exercise. However, local or whole-body vibration and various vibration characteristics might lead to distinct effects regarding the HR regulation of the autonomic nervous system after exercise. Overall, the impact of vibration exercise on the regulation of the autonomic nervous system during the recovery period after intense exercise requires further study.
This study presents some limitations. For instance, we did not examine exercise performance during the recovery period. Some researchers [1, 2] have suggested that the exercise performance during recovery period should be determined when assessing the utility of recovery strategy after exercise. Several studies have also found that despite a lower La, exercise performance during the recovery period did not improve [43, 44]. For example, Sanudo [12] demonstrated that when performing high-intensity exercise until exhaustion, a vibration exercise (25 Hz, 4 mm) during recovery period can improve blood flow in the popliteal artery and prolong the time to exhaustion of the subsequent high-intensity exercise. However, other studies have suggested that vibration exercise during the recovery period after exercise did not improve the removal of blood lactate, and neither increased the time to exhaustion of high-intensity exercise [20], nor the 30-s Wingate test, maximum voluntary contraction, jump height, or sit-and-reach performances [18]. Therefore, although vibration exercise with low-frequency facilitated the removal of blood lactate during recovery period after exercise, further clarification regarding whether this recovery modality facilitates the improvement of exercise performance during the recovery period is required.
CONCLUSION
In conclusion, the results of the present study suggest that low-frequency vibration (8 Hz) can facilitate the removal of metabolic by-products after exhaustive exercise, but has little effect on the modulation of the autonomic nervous system on HR recovery. Vibration exercise has been reported to induce peripheral vasodilation, and produce acute increases in peripheral circulation, blood flow, and skin and muscle temperature [8-12, 18, 39]. Our data also indicate that the improvement in removal of blood lactate during recovery period should occur after the application of low-frequency vibration. These results may help coaches, strength and conditioning professionals, and exercise enthusiasts use low-frequency vibration as part of a cool-down procedure. However, considering that vibration exercise offers numerous operational settings, including various combinations of frequency and amplitude, and local or whole-body vibration, future studies should perform low-frequency vibration exercise as a recovery strategy to improve recovery from strenuous exercise and examine the subsequent exercise performance.
CONFLICT OF INTEREST
The author confirms that this article content has no conflict of interest.
ACKNOWLEDGEMENTS
The authors thank the participants who contributed their time and efforts to undertake this study, and the Polypact International Co. Ltd. who sponsored the consumable materials of Cortex metabolic analysis system. This study is supported by the Ministry of Science and Technology, Taiwan (NSC 98-2410-H-003-123), and by “Aim for the Top University Plan” of National Taiwan Normal University and the Ministry of Education, Taiwan.