All published articles of this journal are available on ScienceDirect.
Effect of Four Weeks Detraining on Strength, Power, and Sensorimotor Ability of Adolescent Surfers
Abstract
Background:
Surfing is a high skill sport that requires a considerable amount of time in a variety of ocean conditions to help develop the fundamental techniques.
Objective:
The purpose of this study was to investigate the effect of four weeks of detraining on strength, power, and sensorimotor ability in adolescent surfers.
Methods:
Nineteen adolescent surfers (13.8 A 1.7 y, 53.6 A 10.8 kg and 165.1 A 8.9 cm) participated in four weeks of detraining (surfing participation maintained but resistance training ceased) following seven weeks of periodized resistance training. Maximal isometric strength, power, and sensorimotor ability pre-test results were determined from the conclusion (post-test) of the first seven-week training block while post-test results were measured at the start (pre-test) of a second seven-week training block.
Results:
Four weeks of detraining significantly decreased the following variables: Vertical jump height by -5.26%, (p=0.037, d= 0.40), vertical jump peak velocity by -3.73% (p=0.001, d= 0.51), isometric strength by -5.5%, (p=0.012, d= 0.22), and relative isometric strength by -7.27% (p=0.003, d= 0.47). Furthermore, sensorimotor ability worsened, with a significant increase of 61.36% (p=0.004, d= 1.01), indicating that athletes took longer to stabilize from a dynamic landing task.
Conclusions:
This demonstrates that surfing, in the absence of resistance training, is not a sufficient training stimulus to maintain physical characteristics. Adolescent surfers with a relatively low training age should avoid cessation of resistance training and strive to maintain consistent resistance training in conjunction with surf training in order to avoid negative decrements in physical characteristics that are associated with surfing performance.
INTRODUCTION
Surfing is a high skill demand sport that requires a considerable amount of time in a variety of ocean conditions to help develop the fundamental techniques. It has been reported that recreational and competitive surfers spend as much as 6.6 ± 4.4 and 12.3 ± 2.8 hours of surfing per week, respectively [20]. In comparison, resistance training has been reported as total durations of 1.5 ± 2.7 and 0.5 ± 0.6 hours per week for recreational and competitive surfers, respectively [20]. With this in mind, resistance training does not appear to be a priority within the surfing community, as more time is spent in the water to improve surfing ability. Anecdotally, surf coaches and adolescent surfers believe spending more time surfing, in the absence of resistance training, is a sufficient stimulus to maintain physical capabilities. Due to the inconsistency of the environment, the priority of the athlete is to surf when the conditions are favourable and resistance train when the conditions are poor [6]. This surf mantra might make it challenging for surfing athletes to be consistent with a periodised resistance-training program because they are busy chasing favourable surfing conditions. Currently, there are no research published on the effects of detraining on strength, power, and sensorimotor abilities in competitive surfing athletes. Therefore, it is imperative to investigate adaptations from resistance training, and then cease training to determine whether surfing in the absence of resistance training is a sufficient stimulus to maintain physical characteristics.
Previous research documented that adolescents participating in a resistance training program not only benefits health and psychological well-being, but also important for growth and motor skill development [8]. Over the years the numbers of adolescents participating in sports has increased, thus increasing the rate of sport related injuries [8]. Therefore, incorporating an appropriate resistance training program under qualified supervisions may be advantagous for reducing the likelihood of sports related injuries [8, 10]. Furthermore, greater physical qualities [e.g., strength and power] have been reported to associate with surfing performance [28, 30]. Recent evidence revealed physical performance reflected selection from a group of elite junior surfers to represent Surfing Australia National Junior Team at the International Surfing Association World Junior Surfing Championship [33]. Collectively, these studies demonstrated the importance of physical qualities for surfing performance and using a group of junior surfers distinguished physical performance among selected and non-selected elite junior surfers. Adequate resistance-training stimulus has been shown to elicit positive neuromuscular adaptations [9,13]; conversely, detraining negatively affects strength and power parameters within a relatively short period [2, 12, 17]. It is important to note detraining [cessation of resistance training] has potential negative implications for athletic performance, whereas a well-planned tapering phase may augment performance variables such as strength, power, and endurance [26]. Previous studies have highlighted decrements in strength and power as a result of detraining following completion of a resistance training program in various populations: children [12], sedentary [2], college-age women [32], and power athletes [16]. However, little is known with regards to the effect of detraining on performance parameters in adolescent surfers.
Faigenbaum et al. [12] demonstrated that children [7-12 y] significantly decreased upper [-19.3%] and lower body [-28.1%] strength over an eight-week detraining period. Izquierdo et al. [17] demonstrated that four weeks of detraining subsequent to 16 weeks of resistance training had a significant negative effect on strength [-9%] and power [-17%] in physically active men. In another study, Staron et al. [32] demonstrated that college-age women significantly decreased leg press [-32%] and leg extension [-29%] strength following 30-32 weeks of detraining. Furthermore, Andersen et al. [2] reported strength, power, and muscle cross-sectional area [CSA] decreased to pre-training levels after three months of detraining in sedentary men. Conversely, Hortobagyi et al. [16] demonstrated power athletes’ strength level did not significantly alter following 14 days of detraining, however, Type II muscle fibre area decreased 6.4%. Interestingly, Santos and Janeira [27] were able to demonstrate that the explosive power was maintained during 16 weeks of detraining in adolescent male basketball players. Collectively, with the exception of Hortobagyi et al. [16] and Santos and Janeira [27], these studies have demonstrated a decrease in strength [2, 12, 27], power [2, 17], and muscle CSA [2, 16], however, it is important to note that different individuals respond and adapt to training stimuli differently. Specifically, the studies by Hortobagyi et al. [16] and Santos and Janeira [27] represent the potential for a population with longer training history to be able to decrease or not experience the decrements in physical capacity seen in populations with lower training history.
Postural control and dynamic balance are critical for the sport of surfing; however, there is a lack of scientific evidence regarding the effect of detraining on sensorimotor ability for adolescent competitive surfers. Drop landing from a pre-determined height followed by a motionless posture is relevant to the sport of surfing as the athletes are required to transition from a dynamic to a static position with the time to stabilization [TTS] currently the accepted test for this ability [36]. This method quantifies the time an athlete takes to stabilize their posture within 5% of body mass upon landing. In addition, this test also quantifies landing force from a vertical direction as this might demonstrate whether detraining affects the ability to effectively attenuate landing forces. Wikstrom et al. [36] reported vertical TTS method established fair reliability with an intraclass correlation coefficient [ICC] measure of 0.78 with a 95% confidence interval of 0.59-0.90. Additionally, drop landing might suggest whether this method is sensitive and valuable to detect small changes of sensorimotor ability for adolescent surfers. For example, during a floater manoeuvre, surfers launch themselves on top of the face of the wave, then drop land to the bottom in front of the broken wave. If the surfer is able to stabilize and control their posture quickly and efficiently, they are more likely to land successfully and transition to the next manoeuvre.
While there is a considerable amount of literature on the positive adaptations from acute and long-term resistance training in young athletes [7, 8, 11-13], it is uncertain whether adolescent surfers are able to maintain physical performance with surfing alone. Therefore, the purpose of this study was to determine the magnitude of changes in physical performance over a four-week detraining period during which the athletes completed no resistance training.
MATERIALS AND METHODS
This study investigated the impact of detraining on strength, power, and sensorimotor ability in adolescent surfers following seven weeks of periodized resistance training. Performance parameters of power [vertical jump height; VJH], maximal isometric strength [isometric mid-thigh pull; IMTP], and sensorimotor ability [time to stabilization during a drop and stick [DS]; TTS] pre-test results were determined from the conclusion [post-test] of a seven-week training block while post-test results were measured at the start [pre-test] of a second seven-week training block. In other words, the four-week washout period between two seven-week training blocks was used to assess the effect of a detraining period. Four weeks cessation of resistance training was based on the participants’ school break schedule. Athletes performed three countermovement jumps to self-selected depth, three IMTP, and five DS trials. The best trial for each variable was used for further analysis.
Participants
Nineteen competitive adolescent surfers with an overall mean age, mass and stature [mean ± SD] of 14.1 ± 1.6 y, 54.0 ± 10.75 kg and 165.0 ± 9.0 cm, respectively, volunteered to participate in this four-week study. Competitive adolescent surfers are defined as surfers who have competed in regional, state, or national competitions. All testing and data management was conducted according to the Declaration of Helsinki and approved by the institutional review committee. Parents and athletes were informed of the risks and benefits of this study and gave consent or assent prior to participation.
Measurements
The performance test session commenced with body mass and stature measurements, followed by a standardized 10-minute dynamic warm-up consisting of body weight squats, knee hugs, thoracic rotation, walkout, duck walk, and lateral shuffle [35]. Prior to the performance test session, athletes were provided details and familiarised with the testing protocols. The athletes then practiced the countermovement vertical jump [CMJ], isometric mid-thigh pull [IMTP], and the drop and stick [DS] testing protocols to reduce possible learning effects. Performance parameters consisted of lower body power [CMJ], maximal isometric strength [IMTP], and sensorimotor ability [time to stabilization during a DS; TTS].
Vertical Jump Performance
The CMJ was used to assess vertical jump height. Athletes were instructed to start in an upright position while standing still on a portable force plate [400 Series Performance Force Plate, Fitness Technology, Adelaide, Australia], which has a built in amplifier and four load cells that measure vertical components of ground reaction force. Prior to data collection, calibration of the force plate was performed as per manufacturer’s instructions. The force data was collected at a sampling frequency of 600 Hz and inverse dynamics calculations were used to calculate peak force as well as peak velocity and jump height based on the impulse momentum relationship as per manufacturer software [Ballistic Measurement System, Innervations, Perth, Australia]. These methods have been previously used in this population [32]. A low-pass 4th order Butterworth filter with cutoff frequencies set at 16 and 10 Hz for velocity, and acceleration data respectively was applied. Displacement and force were not filtered. Athletes were instructed to complete three trials dipping to a self-selected depth while holding a light wooden dowel in contact with the back of their shoulders and then jumping vertically for maximum height [1]. During each jump, if the wooden dowel lost contact with the shoulders, the jump was discarded and repeated. Sixty seconds of rest was provided between jumps. Athletes were encouraged to jump as high as possible and the best jump height was used for further analysis of the following variables: peak velocity, jump height, and absolute and relative peak force.
Isometric Mid-thigh Pull Performance
Maximum isometric strength was measured on a customized mid-thigh pull system with adjustable straps to accommodate individual height differences. Athletes completed three IMTPs with two minutes rest between pulls. Prior to the initial pull, the athletes stood still on the force plate with the shoulders in line with the bar and hands slightly wider than shoulder width apart. They were then placed in a position similar to the second pull of the clean with knee and hip angles corresponding to 130-140° and 140-150°, respectively [15, 18, 29]. In other words, the position of the bar was at a height corresponding to the mid-thigh for each athlete. Athletes were instructed and verbally encouraged to push against the force plate as hard as possible while holding the bar across their mid-thigh with maximal effort for five seconds. Recently, Sheppard et al. [31] have reported excellent reliability [ICC: 0.99 [0.97-0.99] with a percent typical error of measurement of 2.25% in this population. Although Haff et al. [15] used instruction to “pull as fast and hard as possible”, the current authors observed during pilot trials that “push” was the appropriate instruction for this population. The same force plate, personal computer and software used to measure vertical jump performance was used to record and analyse vertical landing force and to derive peak force.
Sensorimotor Performance
Sensorimotor ability was measured via a drop and stick manoeuvre on a portable force plate [400 Series Performance Force Plate, Fitness Technology, Adelaide, Australia] connected to computer software [InnerBalance, Innervations, Perth, Australia] recording landing peak force with a sample rate of 600 Hz. Athletes were given practice trials, however, if additional trials were needed to become competent in the landing task, they were given more. Athletes performed five drop and stick trials barefoot from a pre-determined box height of 0.5 m [34]. They were instructed to step forward off the box with their preferred leg, “land soft” on both feet, and as quickly as possible reach a position with the upper thighs parallel to the ground, similar to the bottom position of a squat. In the event the upper thighs were not parallel to the ground, the trial was discarded and repeated [upper thighs parallel to the ground and holding for three seconds was deem acceptable]. To determine the average of three drop and stick trials, trials with the lowest and highest TTS were discarded. Time to stabilization was calculated from initial contact on landing to the time the athletes stabilized within 5% of body mass. For example, if an athlete’s initial contact occurred at 1.5 s and stabilisation to within 5% of their body mass occurred at 2.1 s, TTS of 0.6 s was recorded. The relative peak landing force [rPLF] was calculated from peak landing force divided by body mass to account for individual differences. In the event the upper thighs were not parallel to the ground, the trial was discarded and the athlete was given another trial. Sixty seconds of rest was provided between each drop landing.
Relative peak landing force was calculated by dividing maximum landing force by body mass. Time to stabilization demonstrated fair test-retest reliability with greater variability shown in relative peak landing force (Table 1).
aSignificantly different from pre test. | |||||
---|---|---|---|---|---|
Measure | Pre | Post | ICC (90% Confidence Interval) | Effect size (d) | Effect descriptor |
VJH (m) | 0.38 ± 0.05 | 0.36 ± 0.05a | 0.78 (0.60-0.90) | 0.40 | Small |
VJPV (m•s-1) | 2.41 ± 0.18 | 2.32 ± 0.17a | 0.88 (0.76-0.95) | 0.51 | Moderate |
rVJPF (N•kg-1) | 20.25 ± 1.95 | 20.62 ± 1.07 | 0.67 (0.43-0.84) | -0.24 | Small |
IPF (N) | 1691.83 ± 430.05 | 1598.79 ± 437.42a | 0.93 (0.86-0.97) | 0.22 | Small |
rIPF (N•kg-1) | 31.51 ± 5.10 | 29.22 ± 4.68a | 0.80 (0.62-0.91) | 0.47 | Small |
TTS (s) | 0.88 ± 0.30 | 1.42 ± 0.71a | 0.72 (0.50-0.87) | -0.99 | Large |
rTTSPLF (N•kg-1) | 4.38 ± 1.62 | 4.03 ± 1.47 | 0.47 (0.19-0.72) | 0.23 | Small |
Training Program
Athletes continued their surf training sessions but did not complete any resistance training throughout the four-week period cessation of resistance training. Prior to this study, the surfing athletes attended resistance training twice a week on non-consecutive days, and completed a seven-week training program [35] consisted of one lower body [LB] explosive exercise [double leg forward jump] and one upper body [UB] explosive exercise [medicine ball slam], two LB strength exercises [overhead squat, dumbbell squat], two UB strength exercises [1-arm row, push-up], and one trunk rotation exercise [medicine ball rotation]. Session two was performed with a similar format consisting of vertical jump, medicine ball chest throw, dumbbell squat then shoulder press, assisted pull up, 1-arm dumbbell row, assisted [straps] single leg squat, push-up and medicine ball woodchop
Statistical Analysis
In order to determine if there were any significant differences from pre- to post-test, pair t-tests were used to compare anthropometry and performance results. All statistical analyses were completed using SPSS for Windows, version 22 software [SPSS Inc., Chicago, IL, USA] with criterion level of significance set at p ≤ 0.05. Cohen’s effect sizes [ES] were calculated as ES=mean change divided by the standard deviation of the sample scores [3]. The magnitudes of the ES’s were evaluated as trivial < 0.2; small 0.2-0.5; moderate 0.5-0.8; or large > 0.8 [5].
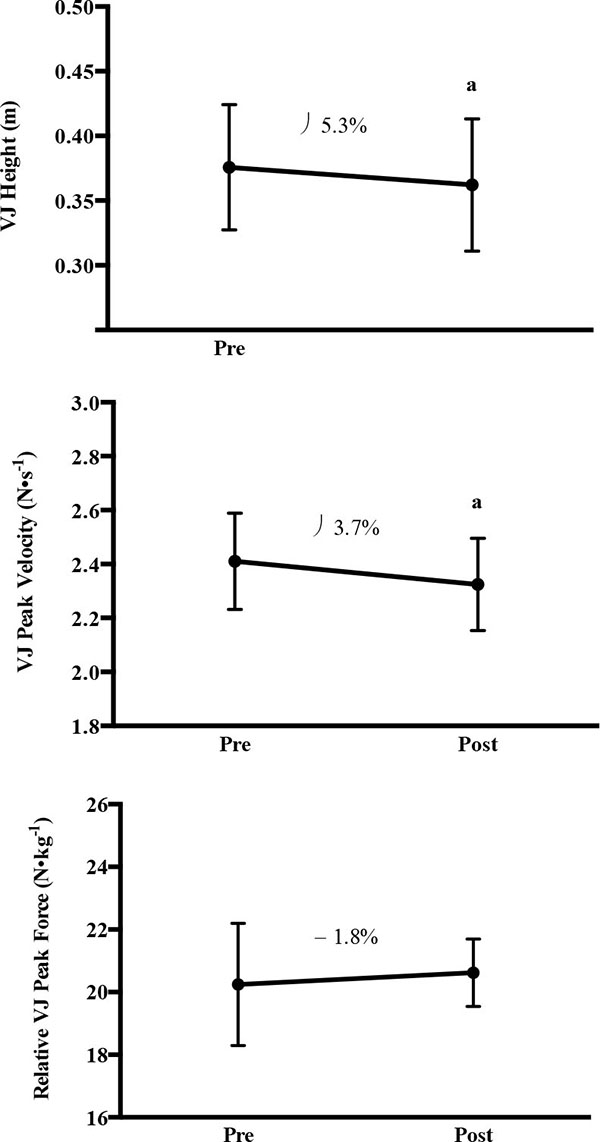
RESULTS
Average strength, power, and sensorimotor ability performance are presented in Table (1) and percent changes for pre to post measures are shown in Figs. (1-3). Power performance measures significantly decreased for vertical jump peak velocity [-3.7%, p<0.001, ES =-0.51], vertical jump height [-5.3%, p=0.037, ES=-0.40], isometric absolute peak force [-5.5%, p= 0.012, ES= -0.-21], and relative peak force [-7.3%, p= 0.003, ES=-0.47]. Sensorimotor ability, via time to stabilization, significantly increased [61.4%, p=0.004, ES=0.99]. In addition, there was no significant change in relative peak landing force during the DS [-2.3%, p>0.05, ES=-0.06]. There was no significant change observed for relative vertical jump peak force [1.8%, p>0.05, ES=-0.06].
DISCUSSION
The purpose of this study was to investigate the effects of a period of cessation of resistance training or detraining on power, strength, and sensorimotor ability subsequent to short-term resistance training. The main finding of this study was that cessation of resistance training for four-weeks negatively affected performance parameters for competitive adolescent surfers. This study provides evidence that surfing, in the absence of resistance training, is not a potent enough stimulus to maintain or increase physical capabilities. Recently, Loveless and Minahan [20] reported that recreational and competitive surfers spend between 2-11 and 9.5-15 hours surfing per week, respectively, while participation in resistance training was reported as 0-2 hours per week. This finding is in line with Mendez-Villanueva et al. [24], indicating that the majority of surfers’ training is performed in the water. The adolescent surfers in our study surfed an average of 12-19 hours per week. It is possible that the higher number of surf hours in the current study might suggest adolescent surfers have more time and access and engage for fun rather than purposeful training in the water.
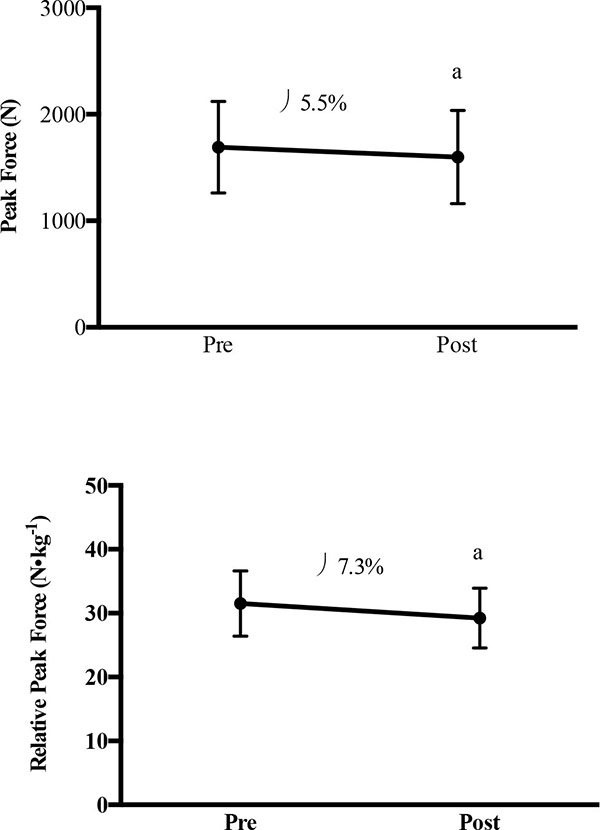
In response to four weeks of detraining, power measures for the vertical jump of peak velocity and jump height significantly decreased by 3.7%, with a moderate magnitude [ES=-0.1] and 5.3%, with a small magnitude [ES=-0.40], respectively. Declines in vertical jump height in the present study are consistent with Faigenbaum et al. [12], who reported a 4.0% decrease subsequent to eight weeks of detraining in adolescents. However, these findings conflict with Santos and Janeira [27], who reported that male adolescent basketball athletes were able to maintain explosive power following 16 weeks of detraining. An explanation for this conflict might be due to a higher volume of plyometrics involved in basketball practice throughout the 16-week detraining period. For example, basketball is a sport that involves continuous use of the stretch shortening cycle during activities such as lay-ups, dunks, rebounding, changes of direction, or blocking shots throughout practice. Although surfers perform similar absorption, braking, and propulsion phase activities during wave riding, the volume is less due to the nature of the sport as wave riding represents only 3.8-8.0% of total activity [14, 22, 23]. Therefore, it might be concluded that higher plyometric volume during basketball practice provides adequate stimulus to maintain power in adolescent basketball athletes compared to adolescent surfers. In another study, Hortobagyi et al. [16] reported that 14 days of detraining did not significantly decrease vertical jump height in power athletes. A possible explanation for this might be that athletes with a longer training age are able to maintain explosive power for an extended time or that acute training cessation was not adequate to induce an effect on explosive power in power athletes.
Lower body isometric strength measures for absolute and relative peak force demonstrated significant decreases of 5.5% [p=0.012] with a small magnitude [ES=-0.21] and 7.3% [p=0.003], also of a small magnitude [ES=-0.47], respectively. These findings are consistent with Faigenbaum et al. [12], who reported that detraining subsequent to eight weeks of resistance training significantly decreased lower body strength [-28.1%] in children ranging from 7-12 years. The magnitude of strength loss in the Faigenbaum study is much greater compared to the present study. Although the duration of their detraining period was double that of the current study, the differences in strength loss are quite large. It has been previously shown that lower physical level athletes have a larger window for adaptation [4]; hence, a possibility for the large decline might be similar for strength losses and gains in younger athletes compared to those with higher physical capacities.
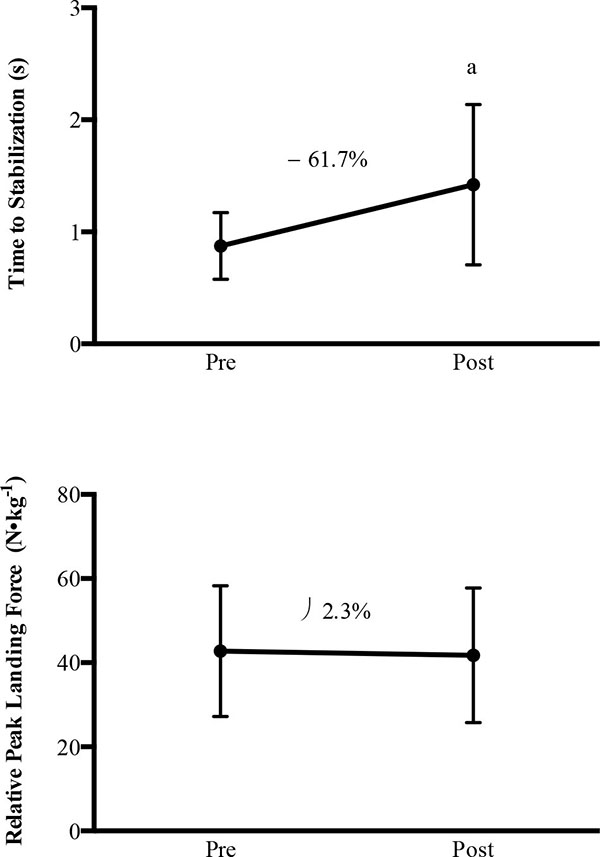
Interestingly, Hortobagyi et al. [16] reported significant decreases in Type II fibre area [6.4%] in power athletes over four weeks of detraining, however, there was no significant decrease identified in lower body strength [-0.9%]. Although it has been documented that muscle CSA is associated with strength gains [21], their findings further support the contention that highly trained athletes are able to maintain their strength following an acute detraining period compared to those with lower strength levels. An interesting finding from the current study is that detraining had a greater effect on muscular strength compared to power output. Similarly, Faigenbaum et al. [12] demonstrated a greater loss in strength than power during eight weeks of detraining. Conversely, Izquierdo et al. [17] reported that Basque ball athletes demonstrated a greater loss in power [-17.0%] compared to muscular strength [-9.0%] after four weeks of detraining. Basque ball involves a diverse of sports played with one’s bare hand, a racket, a wooden bat or basket, against a wall [17]. Collectively, these studies strongly suggest the effect of detraining on power and strength appears to be dependent on training age as well as the duration of detraining. Furthermore, consideration of the type, intensity and volume of physical activity undertaken during the detraining period must also be taken into account regarding the magnitude of changes resulting from detraining [12]. In other words, physical activities such as basketball, soccer, or volleyball place a greater demand on the neuromuscular system compared to activities that limit stretch shortening cycle loading from jumping and landing.
Although surfing is performed in a dynamic environment, the surfer is relatively stable as the speed of the board increases [25]. In response to sensorimotor ability [TTS during drop and stick] following four weeks of detraining, the athletes took significantly [p=0.004] longer to stabilize from a drop landing. However, relative peak landing force was not significantly [p>0.05] altered. Interestingly, strength performance significantly decreased with an associated increase in TTS, however, athletes were able to maintain relative peak landing force.
CONCLUSION
In light of these findings, adolescent surfers experienced a reversal effect when resistance training was discontinued for four weeks. These results demonstrate that surfing, in the absence of resistance training, is not a potent enough stimulus to maintain performance parameters. The results of this study will increase awareness within the surfing community of the deleterious impact of detraining on strength, power, and sensorimotor ability. Competitive adolescent surfers with a relatively low training age should strive to maintain consistent resistance training in conjunction with surf training to avoid the negative decrements in power, strength, and sensorimotor ability, as these are likely to reduce physical capabilities. Therefore, by continuing resistant training in adolescent surfers, training age increases and continued gains in strength, power, and sensorimotor abilities can be continued and therefore more likely to be able to contribute to increased performance during surfing or decreased injury risk and ability to tolerate surfing training loads. Regarding the safety and recommendations for resistance training progressions, junior surfing athletes should seek a qualified strength and conditioning coach and refer to the Strength Training for Young Athletes book for appropriate training intensity, volume and correct exercise technique [19].
LIST OF ABBREVIATIONS
cm | = Centimeter |
CMJ | = Countermovement jump |
CSA | = Cross sectional area |
d | = Cohen’s effect |
DS | = Drop and stick |
ES | = Effect size |
ICC | = Intraclass correlation coefficient |
IMTP | = Isometric mid-thigh pull Methods: first paragraph |
IPF | = Isometric peak force |
kg | = Kilogram |
LB | = Lower body |
m | = Meter |
m•s-1 | = meter per second |
N | = newton |
N•kg-1 | = newton per kilogram |
rIPF | = Relative isometric peak force |
rPLF | = Relative peak landing force |
rTTSPLF | = Relative time to stabilization peak landing force |
rVJPF | = Relative vertical jump peak force |
s | = Second |
SD | = Standard deviation |
TTS | = Time to stabilization |
UB | = Upper body |
VJ | = Vertical jump |
VJH | = Vertical jump height |
VJPV | = Vertical jump peak velocity |
CONFLICT OF INTEREST
The authors confirm that this article content has no conflict of interest.
ACKNOWLEDGEMENTS
The authors would like to thank all the participants and the surf coaches for their time and contributions.