Fatigue and Recovery in Soccer: Evidence and Challenges
Abstract
Background:
Soccer presents physiological, metabolic, physical and psychological demands which can deteriorate players’ performance due to fatigue. The high variability in physiological, metabolic, physical and psychological responses also influences the magnitude of exercise-induced muscle damage, with symptoms negatively affecting neuromuscular function during recovery or subsequent training sessions or matches. Consequently, more precise and consistent knowledge is required in this area to optimize training and performance.
Objective:
Therefore, the purpose is to sum-up current evidence on fatigue and recovery in soccer players, to shed light on factors that can affect players’ performance, and to suggest applications for coaches and further research.
Method:
A comprehensive review of the scientific literature on the field was conducted.
Results:
Physical performance decrements during matches have traditionally been associated with physiological fatigue, but the magnitude of the symptoms in soccer players is unclear and depends on several factors. Moreover, the decline in physical performance during a soccer match is related to specific demands of each match. These could explain inter-individual variability in acute fatigue or training recovery processes when comparing players from the same team. Recovery counteracts the effects of fatigue, both peripheral and central, but there is a lack of consensus about the usefulness of tests used to monitor fatigue and recovery kinetics.
Conclusion:
Although fatigue and recovery in soccer has been extensively studied, there are still uncertainties about the underlying mechanisms because they are influenced by physiological and match-related demands.
1. INTRODUCTION
Soccer players are exposed to mechanical and metabolic stress which might induce physiological changes. Game demands include accelerations, decelerations, jumps, changes of direction, technical and tactical skills to successfully adapt to a rapidly changing environment and achieve optimal performance [1]. There is sometimes a decrease in performance: participation in a single match leads to acute and residual fatigue, characterized by a decline in physical performance over the following hours and days [2]. Fatigue can be described as the inability to maintain a given exercise intensity or power output level [3], although it is usually defined as any reduction in physical or mental performance [4, 5]. The physical demands imposed on soccer players have been widely reported [6-8], but some performance indicators (e.g. total distance covered) represent an inaccurate estimation of the overall physical requirements and the readiness to perform maximally.
Game demands have an eccentric component with a potential exercise-induced muscle damage (EIMD) [1], so the magnitude of EIMD and other physiological alterations elicited by match play are also associated with specific movement demands [9]. Together with a decline in performance, long-term muscle damage and increased levels of intramuscular enzymes and inflammatory markers are reported following soccer competition [9]. There is therefore a relation between EIMD and fatigue: muscle fatigue may partly derive from EIMD [10]. Anyway, soccer players can be fatigued without EIMD symptoms and vice versa.
Recovery counteracts the effects of fatigue, both peripheral and central, and can be defined as the ability to meet or exceed performance in a particular activity [11]. In soccer, the ability to recover from intense training or match is a key aspect of performance [12, 13]: those who recover faster will have a greater advantage [14]. To ensure adequate recovery after soccer matches and training sessions, it is necessary to know the type of fatigue induced and, if possible, its underlying mechanisms [15, 16]. Although there is no consensus on a central strategy for recovery, routine assessments of fatigue and recovery are therefore insightful efforts to ensure that players successfully adapt to training and competition stress and to evaluate athletes’ readiness for return to competition [17].
2. EVIDENCE, MECHANISMS AND CAUSES OF FATIGUE IN SOCCER
Physical performance decrements during matches have traditionally been associated with physiological fatigue [8, 18], but there are different situational variables or contextual factors which can also influence players’ physical performance (explained below). Thus interpreting match-related fatigue is highly complex, although is it clear that fatigue occurs towards the end of matches as well as temporarily during the game, independently of the competitive standard and playing position [19].
Running performance has been deeply analyzed as a fatigue indicator. It has been shown that the distance covered by players can be lower in the second half than in the first half of a game (18% lower) regardless of playing position [20]. In addition, the distance covered performing high-intensity activities can be 20% lower in the last 15 minutes than in the first 15 minutes of the match, regardless of playing position [7]. Furthermore, the total distance covered at different intensities in the first half can significantly influence the distances covered in the second half [8] and can have a significant impact on the recovery immediately following the most intense 5-min periods of the second half [21]. It has been found that there may be a similar difference between the distance covered performing high-intensity running with (148±78m vs. 193±9m, p<0.01) and without ball possession (229±85m vs. 278±97m, p<0.01) between the first 15-min and last 15-min period of the game [7]. Fatigue may also be evidenced by the increased duration of low-intensity activities [22]. Mean recovery time between very high-intensity running bouts can be about 72 s, with a 28% longer recovery time during the last 15 minutes than the first 15 minutes of the game (83±26s vs. 65±20s, p<0.01) [7]. The decline in high-intensity running immediately after the most intense 5-min period can be around 8% [6], and greater in attackers and central defenders, compared to other playing positions [7].
The appropriateness of the commonly used measures of high-speed activity for determining variability in an elite soccer team is debatable. For future research individualization of high-speed running thresholds according to fitness characteristics might be a better indicator of running performance and fatigue occurrence [23]. Additionally, no mean decrement in maximal sprinting speed has been observed during bouts (a minimum of three consecutive sprints with a recovery duration <30 s separating efforts) when up to five consecutive sprints were analyzed, suggesting that considering shorter accelerations could give a greater insight in game-specific situations [24].
Physical performance measurements have also been used as indicators of fatigue, mainly jumping and sprinting performance. Immediately after a 90-min game, fatigue can be evidenced by a reduction of sprint (-3%, p<0.001) [4, 12] and jumping performance (-4.4±0.8%) [12]. These decrements are linked to the players’ profile of soccer activity [25, 26]. Although physical performance declines over the course of a soccer match, it seems that players can be able to maintain their technical abilities in the face of physical fatigue or they adapt a pacing strategy that prevent their skill performance from suffering [27].
The mechanisms that reduce the ability to perform maximally are not exactly understood. It seems that after short-term high-intensity periods in both halves, fatigue is related to disturbances in ion homeostasis within the muscle cell and an impaired excitation of the sarcolemma. In the initial phase of the second half fatigue is linked to lower muscle temperatures compared with the end of the first half, and towards the end of the game fatigue seems likely to be caused by low glycogen concentrations [18]. Fatigue following a soccer match is multifactorial and mainly related to dehydration, glycogen depletion, EIMD and central fatigue [28].
The development of temporary fatigue during high intensity exercise is related to an accumulation of potassium in the muscle interstitium, and not causally linked to high muscle lactate, high muscle acidosis or low muscle creatine phosphate [18]. The accumulation of extracellular potassium can impair the excitation of the sarcolemma (depolarize the muscle membrane potential) and reduce force development [18].
Strength and power decrements towards the end of the game reflect the depletion of glycogen stores in active muscles. In favor of this hypothesis, it was observed in Swedish soccer players that those with a low glycogen content in the vastus lateralis muscle covered 25% less overall distance than the other players [29]. Although muscle glycogen stores are not always totally depleted by the end of a soccer game, large depletion of muscle glycogen have been found [25, 30-32], but they depend on inter-individual differences, such as motivation, physical ability, playing position, etc. Decreases in glycolytic capacity and muscle glycogen are associated with a reduction in blood lactate concentration ([La]) in the second half with respect to the first half, and with the lower distance covered and lower intensity of efforts during the game [19, 33, 34]. The decreased [La] and increased plasma free fatty acid concentrations have been interpreted as a change in the choice of energy substrates [31, 35, 36]. More specifically, changes in the quality and quantity of activities during the last minutes of the game suggest an alteration in the physical capacity of soccer players.
Dehydration and hyperthermia have been suggested as being potentially responsible for acute fatigue in soccer [37]. Soccer players have been reported to lose up to 3 liters of fluid during games in temperate thermal environments and as much as 4-5 liters in hot and humid environments [35, 37], and these are linked to decreases in physical performance. Indeed, a significant correlation (r=0.73; p<0.05) was reported between net fluid loss during the match and fatigue index in a post-match sprint test [38]. In addition, it has been observed that cognitive function is better maintained during 90 minutes of continuous exercise when fluid is supplied to players [39]. Furthermore, a body mass loss of 1-2% can contribute to hyperthermia [40]. During a soccer game, the average core temperature ranges between 39.0 and 39.5ºC [18, 41] whereas muscle temperature has previously been shown to be 0.5–1ºC lower [42]. The hyperthermic state may accelerate the degradation of purine nucleotides and/or catabolism of amino acids with concomitant hyperammonemia, which has been linked to central fatigue [43, 44].
Traditionally, there is relatively little scientific information about the effect of central fatigue on soccer performance. Central fatigue could also be an inherent factor in the game, being important towards the end of the game and linked to the deterioration in physical capacity. It is obvious that central fatigue deteriorates technical performance [45], accuracy and decision-making speed [46], but there is no scientific consensus regarding what is the effect of central fatigue on physical performance [4, 45, 47]. As a consequence, more research is needed to clarify the impact of central fatigue on soccer-specific physical performance.
The physical demands of soccer can induce EIMD [2, 48], leading to strength and power decrements [49-51], increased levels of intramuscular enzymes [2, 50-56], a marked inflammatory response [2, 50, 55, 57, 58] and associated upregulated oxidative stress [2, 48, 54, 59, 60] during recovery. The magnitude of EIMD can vary among athletes, and is greater when a player is not accustomed to the required activity [60, 61], such as in pre-season, transition between seasons, or during the recovery period from an injury. The resulting structural changes may impair physical performance during the hours and days following the match so it is likely a major factor to consider in an attempt to explain post-soccer match fatigue [61]. Anyway, more research is needed because it has also been suggested that running performance is unaffected over prolonged periods of fixture congestion despite the evidence of EIMD combined with a decline in physical performance up to 72 h post-match [62].
Hormonal responses and their relationship to immune function in soccer players have also been associated with fatigue. Soccer matches can cause hormonal stress that induces an increase of substances that can influence leukocytes function, in particular their numerical and functional capacity may be reduced after intense exercise [63-65]. Although such responses to soccer training and competition do not appear to be pronounced, variations in immune cell numbers and function are reported in professional soccer players over the course of a season and could influence soccer players’ performance [63].
Therefore, physiological fatigue is determined by a combination of central (concerned with the descending motor pathways from the brain and spinal cord, which regulate the activity of the muscle cell and energy production) and peripherals (described as impairment within the active muscle) factors [4, 5], and can be higher in a hot and humid environment [38]. The underlying physiological mechanisms of each type of fatigue influence both physical and technical performance, but it seems that physical performance is affected in a greater extent by peripheral fatigue whereas technical performance is influenced by central factors. Anyway, fatigue in soccer players could also be attributed to a combination of contextual factors.
3. SITUATIONAL VARIABLES IN MATCH-RELATED FATIGUE IN SOCCER PLAYERS
The decline in physical performance during a soccer match is not only a direct consequence of physiological fatigue. It seems that professional soccer players regulate their physical efforts according to the specific demands of each game and periods of the game, thus situational variables should be taken into account in soccer performance analysis [22]. It has been suggested that individual activity profiles are highly variable and include elements of self-pacing, because decision making about opportunities to become engaged in play dictates individual activities [66]. In addition, increased player density and congestion, increased intensity of play, and longer stoppage breaks that allow greater player recovery and subsequently more intense play, all determine physical responses of soccer players [67].
The work rate of players can be influenced by the activity profile of opponents. One possible reason could be repetitive pressure from the opposition on an individual player, leading to an incapacity to act in response to game demands [68, 69]. It has also been showed that the total distance covered and extent of high-intensity running during matches can be lower against “weaker” opponent teams than against “better” [8, 70, 71]. Soccer tactics and formation do not influence the activity profiles of players, except for attackers, but affects very-high-intensity running and some technical skills [72]. Physical and technical demands are also influenced by positional interchanges, but players who commonly transition between positions seem able to adapt to these switches [73]. Other factors such as the introduction of substitutes can influence high-intensity running. Indeed, substitutes could cover 15% more distance (p<0.01) performing high-intensity running for a given time period of a match compared to a player who is on the pitch for the entire match [6]. The score-line could also influence physical performance of the team and individual players [74]. It has been confirmed that players perform significantly less high-intensity actions when winning than when losing or when the score is level, because when losing, players are more likely to produce maximal efforts in order to come back in the match [70, 75, 76]. Match location seems to influence the work rate of soccer players too [74]. Some studies showed that home teams cover greater distances than away teams during low-intensity activity [68, 69, 71], but other studies suggest that this parameter is linked to other situational variables (e.g. playing at home and losing against a weak opponent) [75]. The causes of other factors (such as travel effects, referee bias, territoriality, crowd effects, specific tactics, rule factors, and psychological factors) and the way in which they affect performance are still not clear [77].
In conclusion, it is complicated to evaluate the influence of fatigue on the physical performance of soccer players without considering the independent and interactive effects of situational variables related to tactical and strategic factors. Therefore, physical performance should be analyzed according to the situational variables relevant to each game [78, 79].
4. RECOVERY IN SOCCER
Understanding the physiological concept of recovery is essential to avoid the detrimental effects of overtraining. Overall, the recovery process has essentially three phases: immediate recovery (which occurs within a set of exercise, between efforts), short-term recovery (between sets of exercise or interval work bouts), and training recovery (between successive work-outs or competitions) [11]. Immediate and short-term recoveries are linked to metabolic, energy sources and electrolytes regeneration, whereas training recovery is linked to structural and energy sources restoration. In the context of soccer matches or training sessions, these physiological responses are highly variable and depend on several intrinsic factors [1], but they are also influenced by extrinsic factors such as training status, age, gender and muscle fiber type [80, 81]. These could explain inter-individual variability in acute fatigue or training recovery processes when comparing players from the same team.
The activity of most elite soccer players during the competitive season entails 1-week cycles of training, taper, competition and recovery from one week to the next [52]. Furthermore, players from top clubs may have additional commitments such as other cup matches or representing their country in international competitions. In some cases, elite players can have two or three games in just seven days. These competitive demands may impose strains on various physiological systems [64, 82], and can also influence the amount of time needed to achieve complete recovery after a match [83].
Soccer players usually need 48-72 hours to restore metabolic homeostasis, muscle trauma and anaerobic performance decrements [2, 51, 83], although several improvements have been demonstrated 24 hours post-match [25]. Nevertheless, post-game performance recovery and inflammatory adaptations in response to a three-game weekly microcycle (Sunday, Wednesday and Sunday) displayed a different response pattern, with strong indications of greater physiological stress and fatigue after the middle game that was preceded by only a 3-day recovery period [84]. That insufficient recovery time between matches may cause performance deterioration and an increase in the risk of injury [85, 86].
Several authors have analyzed physical performance and injury rates during periods with 2-3 weekly games [68, 71, 74, 85, 87, 88]. Unexpectedly, they pointed out that the total distance covered and distances covered in high intensity running were not influenced by the short recovery period between matches, but instead were determined by situational variables. It must be noted that although a greater difference between halves has been found in the distance covered at high intensity during the second match compared to the first match when they played 2 consecutive matches separated by 3 days, this difference was not significant [87]. It has also been suggested that players are generally able to maintain skill-related performance throughout games and when competing in successive matches within a short time [27]. As a consequence, the analysis of actions that are metabolically taxing and greatly contribute to muscle damage (accelerations, decelerations and changes in direction) during fixture congestion is required for future research [62]. This could help to monitor the recovery status of soccer players.
Sleep provides a number of important psychological and physiological functions but sometimes is not enough to ensure complete recovery [89]. In addition, it has been suggested that reduced sleep quantity and quality and reduced perceived recovery are mainly evident following night matches in elite players [90]. Therefore, recovery strategies after exercise (based on the activity profile of each player) are an essential complement to prepare the next game or training session [52]. The effect of recovery interventions following soccer training is still debatable, due to a lack of studies performed in field situations [91]. For future research it would be interesting to analyze the importance of isolating each strategy and the potential interactions between the techniques [28].
5. FATIGUE AND RECOVERY MARKERS IN SOCCER
In team sports, several markers at rest or during activities with low neuromuscular and metabolic demands are often used [92]. In this context, a change in players’ specific performance is the most relevant marker to differentiate fatigued from recovered athletes [17]. However, most of the field tests used in team sports are physically demanding and induce additional fatigue [1, 93]. Performing these tests at frequent intervals could induce cumulative fatigue and thus alter the recovery kinetics from the initial exercise. Therefore, the battery of tests performed during recovery should be checked to make sure they do not lead to additional fatigue and affect the time course of recovery [1].
Unfortunately, there is a lack of consensus about the usefulness of tests used to monitor fatigue and recovery kinetics in team sport athletes [1, 92, 94]. Several indirect markers (performance, neuromuscular, subjective, and biochemical) are often used [1, 11]. Some markers are not accurate and/or reliable enough to measure muscle fatigue during exercise and its impact on skeletal muscle [95]. Given these difficulties, a variety of other markers should be frequently used in science and practice in order to track the stress and recovery processes [10, 17, 96].
a. Physical Performance Markers
Performance is one of the best markers of fatigue and recovery, because it is one of the most important variables of success in competition [11]. Creating a simple and applicable battery of tests would allow for appropriate alteration of day-to-day training intensities and volumes, maximizing the potential for positive adaptations to training, avoiding overtraining and injury, and more importantly, improving match performance [97]. In any case, there is a lack of noninvasive methods for assessing an individual's level of recovery between sessions [97]. Several tests are usually used to evaluate physical performance of soccer players [98-100]. The order of the tests should also be taken into account: a battery of tests must start with short anaerobic tests (e.g. vertical jump) and end with strenuous tests (e.g. repeated sprints or aerobic performance) [1].
i. Vertical Jump
Muscular power is an important requirement for soccer performance [100] and it is usually measured by jump tests [101-103]. Fatigue impairs jump performance in soccer players [104], thus it could be useful to monitor fatigue. Immediately post-match, countermovement jump (CMJ) performance decreases [12, 59, 105], although several studies did not report significant decrements [106, 107] probably due to a ‘warm-up’ effect [108]. Decreases in vertical jump performance can remain 24 [2, 54], 48 [9, 109] and 72 hours post-exercise [59], so EIMD could play a role in this long-term power decrement. Anyway, 48 hours seems to be enough to return to baseline levels [2, 54]. Therefore, jumps can also be used to monitor the muscle status recovery indirectly. Vertical jump should be assessed during stretch-shortening cycle (SSC) activities, because the complexity of SSC fatigue is well reflected by the recovery patterns of many neuromechanical parameters [110]. Squat jump (SJ) involves a single concentric action, whereas CMJ involves the SSC [111], thus CMJ testing is the most suitable for neuromuscular fatigue monitoring in soccer players and SJ to a lesser extent [1, 112].
ii. Maximal Voluntary Strength
The development of muscle fatigue is typically quantified as a decline in the maximal force or power capacity of muscle [113]. The decline in maximal voluntary strength after a soccer match appears to be a consequence of central fatigue, whereas muscle glycogen depletion, inflammation and muscle soreness per se are the most likely candidates explaining soccer match-induced peripheral fatigue [4, 5]. Consequently, the highest level of central fatigue could be in players with the greatest maximal voluntary contraction decline after the match, whereas players with a large increase of muscle soreness experienced a greater degree of peripheral fatigue [4]. During maximal voluntary contraction evaluation, additional measurements (maximal voluntary activation or M-wave amplitude) could also be analyzed to determine the presence of central fatigue [4]. Several studies have used the maximal voluntary strength of the knee flexors/extensors as fatigue and recovery markers. Although several studies showed no decrement in the strength of these muscles [114-116], the strength of these muscles immediately post-match or specific soccer drills can significantly decrease compared to baseline values, but knee flexors strength decrement seems to be more pronounced than knee extensors strength [4, 5, 12, 52, 59, 107, 117]. Decrements of maximal voluntary strength at 72 hours post-exercise could be related to EIMD [52, 59]. Moreover, the best method for quantifying EIMD is maximal voluntary contraction torque because is accurate and reliable [118].
iii. Sprint and Repeated Sprint Ability
Sprinting performance, an important determinant of physical performance in soccer [1], is decreased immediately post-match but it can be recovered between 5-96 hours post-match [2, 4, 12, 52-54, 58, 59]. That huge difference between studies depends on the high variability of physical performance such as high-intensity running distance during soccer matches, contextual factors, different performance levels, player’s age, or training status. A relationship exists between central fatigue indicators and sprint performance decrements. A 48-hour reduction in voluntary activation was observed after an elite soccer match with a significant relationship (r=20.63; p=0.01) between sprint performance decrement and maximal voluntary activation immediately after the match [4]. Moreover, the reduction in voluntary activation may be because of inhibition caused by muscle soreness [119] so changes in sprint performance during recovery period would have a rather peripheral impact than central fatigue [53]. As a result, it is a practical tool to monitor fatigue or recovery status.
Repeated Sprint Ability (RSA) can also be used after a match (to monitor match-related fatigue), or during recovery period (to identify players who are not able to cope with the intermittent demands of soccer). Indeed, significant correlations have been found between RSA mean time and the distance covered in very high-intensity running (>19.8 km/h) (r=-0.60, R2=0.36; p<0.01) and sprinting (>25.2 km/h) (r=-0.65, R2=0.42; p<0.01) during official matches [120]. RSA tests are physically exhausting, which may explain the lack of studies with RSA tests during the recovery period compared to studies with single sprints. So, using RSA tests with few sprints is suitable during the recovery process [42, 106], but they should include changes of direction, a characteristic movement pattern of team sports [121]. However, the construct validity of classic tests of RSA in soccer is still debatable due to the various data available from time-motion analyses of sprints during games [24].
iv. Aerobic Performance
Soccer is an intermittent sport in which the aerobic energy system is highly taxed [30]. Players use their physical capacity during competitive games, so aerobic performance assessment can be useful to monitor post-match related fatigue. A game induced impairment in intermittent exercise performance can occur, and the type of fatigue that occurs in the late stage of a match has an impact on recovery capacity during sustained intense exercise bouts interspersed by short recovery periods [106]. Further research is necessary to assess aerobic fitness during recovery using protocols such as RSA tests [122-124] because aerobic performance measurements can induce fatigue and influence the recovery time course of anaerobic markers [1].
b. Skill Performance
Successful players perform better than others in skill-related actions, such as passing, shooting and dribbling [125, 126]. Technical skills could be deteriorated following high-intensity and localized muscle fatigue [127], but players seems to be able to preserve their technical abilities during and after a match [4, 27]. Therefore, skill performance may not be a reliable tool to detect fatigued players after a soccer match.
c. Subjetive Markers
Coaches can use objective (performance, physiological, biochemical) and subjective measures for athletes’ monitoring. Athletes can monitor their physiological stress during exercise as well as retrospectively provide information regarding their perceived effort post training or competition [95]. The perception of effort can be quantified by rating of perceived exertion (RPE) [128], a valid marker of exercise intensity [129] and a useful tool in the control of training load, exercise prescription and exercise monitoring [130, 131]. It can be used separately for respiratory (RPEres) and muscular (RPEmus) aspects [132, 133]. Consequently, this method is used extensively in soccer [53, 132-134]. Athletes are able to differentiate between perceived effort and other sensations, such as muscle pain, muscle tension, muscle discomfort or wellness (fatigue, vitality, sleep quality), so it can be a helpful non-invasive assessment of the fatigue-recovery cycle [1, 135, 136]. As a consequence, several questionnaires are also used in high-performance sport programs, such as Total Recovery Scale (TQR), Recovery-Stress Questionnaire for Athletes (REST-Q-Sport), Profile of Mood States (POMS), and Daily Analysis of Life Demands for Athletes (DALDA). Sometimes consistent associations between subjective and objective measurements have not been found [137] and athletes could manipulate data and/or over- or under-estimate training load [95]. Thus, subjective information may need to be corroborated with physiological data [138].
d. Cognitive Markers
A soccer match involves mental activities for both goalkeepers and outfield players [139], and mental fatigue has been shown to impact cognitive and physical performance [140, 141]. Neurocognitive mechanisms underlying the effects of mental fatigue are still poorly understood, although scientific evidence pointed out that mental fatigue is associated with lower cognitive performance and inadequate performance adjustments after errors [142]. Therefore, studies focusing on the assessment of cognitive markers during recovery are necessary to investigate the underlying component of mental fatigue in this context [11, 83]. For instance, tasks of psychomotor speed (reaction time, motor time, response accuracy and speed, short-term memory task, etc) are relevant parameters to assess in the detection of fatigue or altered recovery-stress balance [140, 143].
e. Biochemical Markers (Biomarkers)
A biochemical, biological or muscle-status marker (biomarker) is a measurable product or substance used as an indicator of a biological state, to objectively determine the body’s physiological or pathological processes [144]. Soccer causes an increase in metabolism with significant hematological changes [96, 145], thus information from biomarkers could be used to optimize training adaptation, training load, and match participation [83, 145, 146]. Biomarkers responses are influenced by several factors and reflect multiple interactions, so interpretation must be cautious [147]. To date, no single biomarker has been validated for daily routine [95]. As a result, several biomarkers must be considered together to understand the metabolic and physiological mechanisms underlying physical stress in soccer [10, 17, 83, 96]. Some of the most well-known biomarkers of fatigue are those originating from adenosine triphosphate (ATP) depletion or from immunology [10]. Considering that muscle fatigue may partly derive from EIMD [10], muscle damage biomarkers such as creatine kinase (CK), myoglobin (Mb) and lactate dehydrogenase (LDH) and those related to inflammation, such as blood leukocyte count, interleukin-6 (IL-6) and C-reactive protein (CRP), could also be useful [148, 149].
To which extent a shortage in ATP contributes to muscle fatigue is still currently under debate, but it has been shown that a decrease in available ATP at specific locations in association with the function of transport systems may contribute to the development of muscle fatigue [150]. Depletion of ATP, or increase in adenosine diphosphate (ADP), may contribute to muscle fatigue, thus biomarkers from the metabolism of ATP ([La], ammonia, and oxipurines) could be used to monitor acute fatigue [150]. [La] appears to be a meaningful biomarker of muscle fatigue if workload conditions are standardized [151]. Soccer is a sport consisting of high-intensity intermittent exercise, and [La] correlates with the magnitude of exercise performed immediately before blood sampling [152]. As a result, increases in [La] in soccer players must be used carefully as a biomarker of fatigue. Another marker to monitor metabolic activity, energy requirement and the onset of muscle fatigue is blood ammonia, which closely follows [La] response [151]. Ammonia increases after a soccer match [153, 154] and could have detrimental effects on fatigue-related performance, thus particular attention should be paid to their monitoring [153].
ATP can be synthesized by anaerobic or aerobic glycolysis, amongst others. In relation to muscle glycogen, urea concentration is a useful biomarker to evaluate athletes’ level of fatigue and adaptation to training [155, 156]. Urea levels are highly variable among players due to dehydration [38], increased cyclic nucleotides and amino acid breakdown [157], or insufficient level of physical demands during soccer matches to induce significant changes in urea concentration [158]. At any case, more rest is needed if 24 hours post-exercise blood urea has not return to normal baseline concentration [159]. Limited information exists on the characteristics of elite-level players, but most studies have detected significant increases in post-match urea concentrations [57, 158, 160]. However, no significant changes in urea levels have been reported between periods of high and low competitive density during a season [161]. So more research is needed to understand the fatigue-recovery cycle and help coaches to improve individual training prescription.
Associated to immunological responses, monitoring hormonal parameters during professional soccer training could be useful to evaluate accumulated fatigue, overreaching and overtraining [162]. In this way, hormonal responses depend on many parameters, such as exercise duration and intensity, adaptation to exercise, homeostatic requirements, emotional and physical tension, ambient temperature, oxygen availability, and fatigue [157]. This may have an influence on the substantial fluctuation of testosterone (T) levels over a season, but an appropriate training program should not involve T declines that reflect the accumulation of fatigue throughout the season [162-165]. T decrement can also be detected after a match, so those players with smaller decreases in T concentration show lesser decrease in their immune function [166]. Nonetheless, conflicting results have been found in T responses after a soccer match [2, 56, 167], probably due to factors such as location (home versus away), opposition standard (i.e. strong or weak team) [168], and match result [169]. Such as T, a large variability has also been found on cortisol (C) levels during the competitive seasons [162]. Training load, pressure to achieve results and adverse environmental conditions could be responsible for midseason increases found in some studies [165]. However, it is evident that post-match C levels are significantly greater compared to pre-match in semi-professional players [56], and they return to baseline levels between 24 [2] and 48 hours post-match [55]. When full competitive matches were played on consecutive days, C concentrations could be reduced, but additional research is needed to confirm this hypothesis [170]. To date, C may be used to monitor recovery processes and fatigue, even for short time periods [50, 171]. Similarly, the ratio between the concentration of T and C (T/C ratio) is frequently used as indicative of the stress level imposed by the exercise: a decrease in T/C ratio reflect catabolic stress. Even so, a decreased T/C ratio does not automatically influence team performance decrements or team overtraining [96] because T/C ratio also shows significant changes during a competitive season, with significant increases at the end of pre-season and decreases at mid-season [162, 165]. It has been suggested that, in preseason, players are not fatigued and can respond adequately to the training demands (T/C ratio values reflect these adaptations), whereas mid-season values are not associated with overtraining syndrome but instead are linked to the volume and intensity of previous working loads (amount of competitions), the inability to adapt and/or hormonal dysfunction [162]. Although, no difference was seen in T/C ratio from pre-match to post-match in semi-professional soccer players [56], others authors pointed out that T/C ratio can decrease by 64.2% after the match [166], and may stay low until 48 hours post-match [55]. The published results confirm that T/C ratio is highly variable [172]. Consequently, the variation in the relative free T/C ratio to baseline is a better method for studying and preventing overtraining syndrome in comparison with traditional interpretative schemes (decrease >30%) [173].
Measurements of blood CK, LDH and Mb are commonly mentioned in scientific literature to detect EIMD [174]. Post-match increases in plasma CK have been observed [158], and are smaller when pre-match levels are greater [175], but may go up to 84% (p=0.17) when pre-match levels are in the normal ranges [56]. The majority of studies showed high concentrations of plasma CK at 24 [9, 53, 176], 48 [2, 50, 54, 55, 177], and 72 hours post-match [52]. Post-match increases in LDH have also been reported in elite and sub-elite female teams (p<0.01) [167], which can be greater 48 hours post-match [2] and maintained 72 hours post-competition [2, 52]. Moreover, Mb increases from pre-match to post-match could also remain until 72 hours post-match [2, 52, 55]. Therefore, it seems that muscle damage biomarkers response follows the same pattern. Among these substances, CK is considered the best indicator of a breakdown in muscle cell structure [174]. However, the substantial individual variability in CK responses to the same exercise bouts could reduce the usefulness as a biomarker of players’ recovery status [178].
Inflammatory processes are induced during exercise as a result of muscle metabolism and EIMD. The most frequently reported biomarkers of the inflammatory response include blood leukocyte count, IL-6 and CRP [148]. IL-6 increases immediately after a soccer match, but drops rapidly to pre-match levels and returns to baseline 24 hours post- match [2, 60, 176], whereas neutrophil counts can remain significantly elevated 48 hours post-match [50] and CRP peak may occur between 24-48 hours post-exercise [55, 58]. As a result, it can be confirmed that a single soccer game induces marked but transient inflammatory responses and clearly indicates the need of enough recovery for elite soccer players after a match [2].
In any case, these measurements are not convenient for repeated field measures in players, and improvement in these markers seems much less useful if performance is not improved [11]. Biomarkers measurements should not replace performance testing, and they might be used together to provide additional information about the underlying physiology [179].
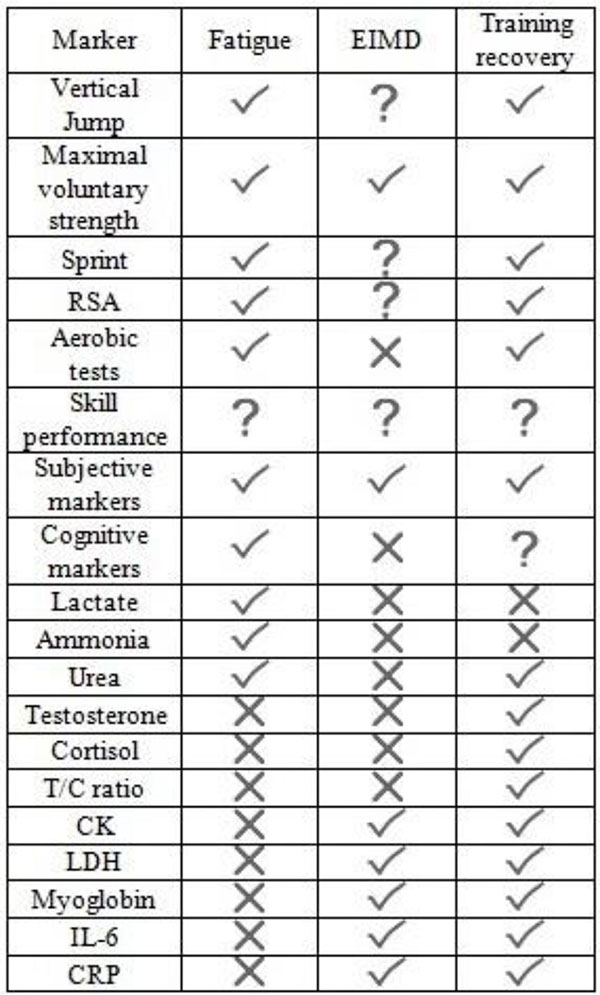



CONCLUSION
Soccer players experience high physiological stress during an exhausting soccer match or training session. These physiological changes result in fatigue which can decrease performance. Moreover, physical performance decrements are also influenced by the specific demands of each game. This may explain the high variability in the metabolic, physiological and psychological responses of players during soccer matches or trainings. The magnitude of EIMD can also be different according to the requirements of each game and players’ characteristics and it could explain inter-individual variability in recovery processes in players of the same team. From a practical point of view, the variability of the responses amongst players leads us to recommend an individual analysis of the results. To assess the readiness of soccer players to put up with the high demands of soccer, researchers have used several markers, but there is a lack of consensus on the usefulness of some of them. Therefore coaches must use markers with high accuracy and combining, at least, one performance, one cognitive or subjective, and one biochemical marker. The analysis of this review may therefore provide important information to coaches, help them to design training schedules and improve individual training prescription, to monitor the recovery status of soccer players, and to adjust players’ recovery strategies.
LIST OF ABBREVIATIONS
ATP | = Adenosine triphosphate |
ADP | = Adenosine diphosphate |
C | = Cortisol |
CK | = Creatine kinase |
CMJ | = Countermovement jump |
CRP | = C-reactive protein |
DALDA | = Daily Analysis of Life Demands for Athletes |
DJ | = Drop jump |
EIMD | = Exercise-induced muscle damage |
IL-6 | = Interleukin 6 |
LDH | = Lactate dehydrogenase |
Mb | = Myoglobin |
POMS | = Profile of Mood States |
REST-Q-Sport | = Recovery-Stress Questionnaire for Athletes |
RPE | = perceived exertion scale |
RPEmus | = Musculature perceived exertion scale |
RPEres | = Respiratory perceived exertion scale |
RSA | = Repeated sprint ability |
SSC | = Stretch-shortening cycle |
SJ | = Squat jump |
T | = Testosterone |
TQR | = Total Recovery Scale |
T/C | = Testosterone/cortisol ratio |
[La] | = Blood lactate concentration |
CONFLICT OF INTEREST
The authors have no support or funding to report, and declare they have no conflict of interest related to the content of this comprehensive review.
ACKNOWLEDGEMENTS
Declared none.