All published articles of this journal are available on ScienceDirect.
Exhaustive Classification and Review of Techniques and Research Program for Techniques for Skate Skiing, Classical Skiing, and Ski Mountaineering
Abstract
Background:
Scattered analysis of some cross country skiing techniques is present in the literature.
Objective:
To provide an exhaustive classification and systematic overview of techniques in cross country skiing.
Method:
This paper classifies six techniques for skate skiing (gliding diagonal skate skiing G1, paddling G2, double dance G3, two skate G4, marathon skate, combiskate G5), four techniques for classical skiing (running diagonal stride RUN DS, diagonal stride DS, double poling kick DK, and double poling DP), five techniques for both skate skiing and classical skiing (herringbone HB, side-stepping G6, low tuck G7, plowing, skidding), and five techniques for steep terrain ski mountaineering. Classification occurs according to degrees of inclination of the terrain, speed ranges, whether the technique is propulsive or for downhill velocity control/turning, frequency of time applied, and literature references. Furthermore, techniques depend on snow conditions, friction, competition, training, wind, positioning within a race, the skier’s expertise, exhaustion level, acceleration, deceleration, etc.
Results:
Techniques can influence who wins races in cross-country skiing. Reasons for technique changes are provided, exemplified with technique changes through terrains with different inclines. Literature references are given for inclines and speeds for the various techniques.
Conclusion:
Essential for maturing skiers are the frequency and quality by which the six techniques for skate skiing, four techniques for classical skiing, and five techniques for both styles except mountaineering, are trained, when to use them, how and in which sequence they are introduced, and adequate supplementary training (e.g. crunches for double poling DP). A research program is sketched.
1. INTRODUCTION
In both competitive and recreational cross-country skiing, and ski mountaineering, skiers change between different techniques. Knowing which techniques are available, and being able to choose the correct technique, is essential for both performance and the pleasure of skiing. It is of interest to study the mechanisms behind technique changes. This paper classifies techniques in skiing. Technique classification is useful for elite skiers to increase awareness of which techniques are actually used, and which are efficient and optimal under which circumstances. Technique classification is also useful for regular skiers to realize which techniques deserve to be trained to enhance performance, for ski mountaineers, for skiers in the military, for adventurers on strenuous exhibitions lasting weeks or months in challenging environments, for skiers involved in skiing as recreation, to avoid injuries, and for the general public to gain insight into skiing.
Early use of the notion of techniques in skiing was made by Nilsson et al. [1], using a car gear analogy. Skiers switch between techniques depending on changes in incline, speed, and many other factors. The various techniques constitute a gear system. A low speed technique or low gear is used on steep inclines, while higher speed techniques or higher gears are used on the flat. Stöggl et al. [2] considered techniques as involving different patterns of motion.1 The gear analogy has also appeared in recent literature [3-7], without providing a systematic or exhaustive overview. Whereas gears in cars, motorbikes, bicycles, and various machineries are mechanical, the techniques in skiing are produced biologically by the human body using various equipment, such as skis, poles, wax, clothing, etc. As a skier proceeds from uphill to downhill, as the speed increases, etc., the natural progression through, or switches between, the various techniques, makes it informative to refer to these changes as shifts from low speed to high speed techniques. Thus the term technique refers to various patterns of motion, and low versus high speed couples the technique to an ordinal speed scale where the technique applies.
Since cross-country skiing competitions take place on varying terrain and at widely varying speeds and external conditions (e.g., snow structure, air temperature and humidity), skiers frequently change between different techniques. This is a unique aspect of cross-country skiing compared to most other sports. Kvamme et al. [4] suggested that the decisive factor in skiers’ selection of which technique to use is the level of incline. Typically, low speed techniques are applied uphill, and high speed techniques are applied in easier terrain such as flat and downhill terrain at higher velocities, or under low friction snow conditions [3]. Andersson et al. [3] found that skiers’ technique selections are not only determined by incline, but also by skiing speed, the length of the uphill slopes and the skiers’ fitness level.
Technique changes are made for many reasons. First, different techniques use the upper and lower body muscles differently. Second, the lower body is used in uphill diagonal skiing, and can rest on flat terrain while using double poling. Similarly in skate skiing, uphill skiing requires using the legs, which can rest downhill where other muscle fibers in the legs are used. Technique changes further depend on the skier’s fitness level, such as the ability to produce power by the upper and lower limbs, ski and snow characteristics, body height and mass, as well as tactical aspects and individual preferences based on earlier experience.
Cross-country skiing is divided into skate skiing and classical skiing. This paper conducts a scrutiny and thereafter classifies techniques for skate skiing and classical skiing, including ski mountaineering. Although in ski mountaineering different equipment, i.e. special skis with climbing skins, grips, special bindings, boots, and poles, are used, mountaineering techniques are also used by skiers with regular cross country skis engaged in recreation, expeditions, etc. Some skiers include climbing skins, and sometimes additional mountaineering equipment, in their backpacks to be attached to or combined with regular skis.
The various techniques are used in different degrees. A handful of techniques may be used in the most common skiing tracks, while others are used in ski mountaineering. Realizing which techniques actually exist is useful, just as it is useful for a cyclist or driver to know the number of gears on one’s bicycle or car. Perhaps a technique exists that has not been explored. This is useful for outsiders who want a technique overview e.g. to determine which techniques to train and avoid. And it is useful for insiders to determine which techniques to train under which circumstances to maximize various objectives.
The techniques are positioned into a systematic structure ranging from steep uphill to steep downhill terrain. To the authors’ knowledge this has not been done earlier. Earlier research has confined attention to the techniques used from moderate uphill to moderate downhill terrain. The techniques for steep uphill and steep downhill movement are rarely used in competitive skiing, but are to some extent used in recreational skiing, on strenuous exhibitions, in the military, and for work-related purposes. We stipulate the degrees of inclination, speed, and the percentage time where these techniques are employed, laying the groundwork for subsequent empirical work.
Many of the cited articles have a certain format. Either one or a few techniques are chosen and described. A certain
1 They developed an automatic algorithm applyng a mobile phone to identify skate skiing techniques.
number of participants are recruited to perform certain tasks involving the technique(s). The characteristics of the participants and how they perform their tasks are reported. The results and implications are analyzed and discussed, and conclusions are provided. Future research possibilities are sometimes sketched. This is all well and good. However, the authors of the current paper believe that from time to time a research community may benefit from choosing a different approach and format. In this paper this is done, first, by scrutinizing all potentially possible techniques. Second, realizing that research within the community evolves in multifarious directions, often not known by more than each individual researcher or group of researchers, this paper attempts to flesh out a description of planned future research. Such a description seems especially needed for a paper analyzing not only one or a few techniques, but all imaginable techniques. The sketched description of future research may or may not overlap with each individual researcher’s view of future research possibilities. Through reflection, discussion, and peer review of the many research possibilities, future research may develop fruitfully benefiting everyone.
Although techniques are well known in the literature, and several have been studied individually, a systematic overview of techniques is lacking, which is the purpose of this paper to provide. Lacking are also accounts of technique changes, and systematic overview over which factors impact how and why they are made. Some of this we attempt to provide, and we indicate research possibilities. This paper is theoretical and conceptual, systematizing some research that has been made, and paving the ground for future empirical research on specific techniques and technique changes. No participants have been recruited, and no experiments with measurements have been made. Future research should compile empirical data for the various individual techniques and technique changes more exhaustively and systematically. Science advances by moving back and forth between the theoretical domain (domain of justification) and the empirical domain (domain of discovery). This paper operates mainly in the theoretical domain, but delves into the empirical domain by sketching a program for future empirical research.
The information concerning characteristics of techniques is compiled through research experience, literature review, and personal skiing experience applying the techniques in various skiing expeditions. As supplementary methodological quality control of the considered literature, the title field in the Web of Science database was required to have the letter combinations “ski”, “cross”, and “country”, and additionally either “technique” or “gear”, which gave 31 hits February 13, 2017. (The letter combination “gear” appeared only once, and that title also contained the word “technique”.) Additionally, searching with “marathon” and “skate” in the title field gave one relevant hit, i.e. Gervais and Wronko [8].
Section 2 presents materials and methods for the techniques in skate skiing and classical skiing. Section 3 presents results divided into technique changes in skate skiing and classical skiing. Section 4 discusses and suggests a research program for technique changes. Section 5 concludes.
2. MATERIAL AND METHODS
Tables 1 and 2 classify, for skate skiing and classical skiing, respectively, the techniques employed, with hypothetical degrees of inclination for the terrain where each technique is used, tentative speed ranges, whether the technique is propulsive or for downhill velocity control/turning, and hypothetical frequency of time applying each technique by trained professional skiers in typical tracks seen in competition.
Techniques | D | S | PV | F | Literature |
---|---|---|---|---|---|
Sideways uphill movement | 45≤x≤90 | <3 | P | R | |
Upward zigzagging | 10≤x≤60 | <3 | P | R | |
Uphill herringbone HB | 10≤x≤30 | <3 | P | O | Andersson et al. [11], Smith [12] |
Gliding diagonal skate skiing G1 | 8≤x≤20 | <5 | P | C | |
Paddling G2 (V1) | 3≤x≤10 | <10 | P | C | Myklebust et al. [37], Boulay et al. [13], Kvamme et al. [4], Nilsson et al. [1], Smith et al. [38], Bilodeau et al. [28], Bilodeau et al. [39], Hoffman and Clifford [40], Mendia-Iztueta et al. [41], Smith [42], Smith [12], Andersson et al. [3] |
Double dance G3 (V2) | -2≤x≤4 | <10 | P | V | Stoggl et al. [43], Moxnes et al. [9], Myklebust et al. [37], Sandbakk et al. [44], Boulay et al. [13], Kvamme et al. [4], Nilsson et al. [1], Ohtonen et al. [45], Ohtonen et al. [46], Mikkola et al. [47], Bilodeau et al. [28], Bilodeau et al. [39], Hoffman and Clifford [40], Karvonen et al. [48], Mendia-Iztueta et al. [41], Andersson et al. [3], Grasaas et al. [32] |
Two skate G4 (V2A) | -2≤x≤2 | <10 | P | V | Boulay et al. [13], Nilsson et al. [1], Bilodeau et al. [28], Bilodeau et al. [39], Andersson et al. [3] |
Marathon skate | -2≤x≤2 | <10 | P | O | Gervais and Wronko [8], Hoffman and Clifford [40] |
Combiskate G5 | -5≤x≤0 | <10 | P | V | Andersson et al. [3], Nilsson et al. [1], Bruzzo et al. [49] |
Side-stepping G6 | -10≤x≤0 | >0 | PV | C | Sandbakk et al. [20], Bucher Sandbakk et al. [19], Andersson et al. [3] |
Low tuck G7 | -10≤x≤0 | >2 | PV | C | Andersson et al. [3] |
Plowing | -20≤x≤-10 | >0 | V | O | Sandbakk et al. [20], Bucher Sandbakk et al. [19] |
Skidding | -20≤x≤-10 | >0 | V | O | Sandbakk et al. [20], Bucher Sandbakk et al. [19] |
Downhill backwards herringbone HB | -30≤x≤-10 | >0 | V | R | |
Downhill zigzagging | -60≤x≤-10 | >0 | V | R | |
Sideways downhill movement | -45≤x≤-90 | >0 | V | R |
The hypothetical degree of inclination or slope between the tangent of the track and the horizontal level is expressed as x, see e.g [9] and [7]. That is, slope angle x=Arctan(vertical distance/horizontal distance). For small angles x we have Tan(x)≈Sin(x)≈x when x is measured in radians. 45 degrees written as 45° means π/4≈0.79 radians. The studies referenced in this paper report their results in degrees, common in the literature, except Sandbakk et al. [10] reporting incline as percentage, i.e. %, which also occurs. The slope percentage is measured as 100(vertical distance/horizontal distance). The theoretical outer limits for incline x are -90≤x≤90 measured in degrees and -∞≤x≤∞ measured in percent, where 90 means vertical uphill 90 degrees, and -90 means vertical downhill 90 degrees. The uphill incline of the last 3 km of the Tour de Ski ski competition is 9.46°. Uphill skiing with skins is possible at 20-30°. Above 55° incline, standing with parallel skis is challenging.2 Although 90° uphill, i.e. uphill movement along a vertical wall, is impossible for a skier, we keep this extreme degree as an outer benchmark since any degree below 90 is arbitrary and may change through new technology, new skills, etc. Skiing downhill steeper than -38° is challenging, though a few have skied steeper than -80°.3 For simplicity, we also keep the outer limit of -90° downhill, which usually means that the skier falls vertically due to gravity. Lower angles are arbitrary and may change through new technology, new skills, etc.
2 The most efficient angle for skinning for uphill skiing by trained athletes is 13-16°, http://www.skintrack.com/skimo-racing/vertical-speed-skimo-uphill-running-slope-angle/ . Uphill skiing with skins can be done at 20-30°, http://outdoors.stackexchange.com/questions/7686/how-steep-of-a-slope-can-you-skin-up-in-at-gear, retrieved February 15, 2017. Above 55° standing with parallel skis becomes challenging since the downhill leg is extended which the uphill leg is flexed, http://www.sierradescents.com/technique/2015/05/05/steep-skiing-uphill-ski-strategies.html, retrieved February 15, 2017.
3 Skiing down a modern staircase, about -38°, is too steep for most people, but is the incline of the groomed slope in Harakiri—Mayrhofen, Austria, http://www.thedailybeast.com/galleries/2010/12/21/the-world-s-13-most-dangerous-ski-runs.html, retrieved February 15, 2017. One example of steeper downhill when the minimum section length is 300’ is Corbet’s Couloir (Jackson Hole, WY) at -53°, http://www.skibum.net/do-it-up/comparing-steepness-of-ski-trails/ . Top alpinists may operate at -60--65°. It is indicated that -75° is the greatest slope at which snow can stick to the slope, http://www.skiingmag.com/uncategorized/how-steep-is-steep, retrieved February 15, 2017, which depends on a variety of factors. Some skiers have skied down steeper than -80°.
A propulsive technique is defined as applying locomotive power to move the skier forward, or at least not resisting forward movement. In contrast, a downhill velocity control/turning technique means that locomotion is provided by gravity which the skier seeks to control. The hypothetical frequency of time of application is expressed as very common, common, occasional, and recreational/mountaineering. These hypothetical values should be tested in future research.
How much different techniques are used by skiers is influenced, as partly mentioned earlier, by factors such as performance level, effort level, strength, power, biomechanics, the skier’s physiological and psychological factors, prior utilization of the various muscles, exhaustion, nutrition, terrain/course, wind, temperature, humidity, snowing/raining, if racing is performed on snow vs. roller skiing, etc. Whether gender impacts technique selection should be scrutinized in future research.
Techniques | D | S | PV | F | Literature |
---|---|---|---|---|---|
Sideways uphill movement, Upward zigzagging, Uphill herringbone HB are as in Table 1. | |||||
Running diagonal stride RUN DS | 8≤x≤20 | <5 | P | C | Lindinger et al. [22], Stoggl et al. [24], Björklund et al. [29], Andersson et al. [50], Björklund et al. [51] |
Diagonal stride DS | 3≤x≤10 | <10 | P | V | Stoggl et al. [43], Lindinger et al. [22], Kehler et al. [23], Stoggl et al. [24], Moxnes and Hausken [30], McGawley and Holmberg [52], Nilsson et al. [1], Pellegrini et al. [5], Pellegrini et al. [36], Sandbakk et al. [53], Fasel et al. [54], Schwirtz and Lagerstrom [55], Bilodeau et al. [28], Bilodeau et al. [39], Hoffman and Clifford [40], Karvonen et al. [48], Mendia-Iztueta et al. [41], Smith [42], Smith [12], Carlsson et al. [34] |
Double poling kick DK | -2≤x≤4 | <10 | P | V | Göpfert et al. [26], Nilsson et al. [1], Pellegrini et al. [5], Pellegrini et al. [36], Hoffman and Clifford [40] |
Double poling DP | -2≤x≤2 | <10 | P | V | Stoggl et al. [43], Björklund et al. [29], Choi and Ryu [56], Danielsen et al. [57], Faiss et al. [58], Hauser et al. [59], Holmberg et al. [27], Holmberg et al. [60], Knight [61], Nilsson et al. [1], Pellegrini et al. [5], Pellegrini et al. [36], Rud et al. [62], Sandbakk et al. [44], Stoggl and Holmberg [63], Stoggl and Karlof [64], Stoggl et al. [24], Wiggen et al. [65], Zoppirolli et al. [66], Zoppirolli et al. [67], Sandbakk et al. [53], Mikkola et al. [68], Lindinger et al. [69], Hoffman and Clifford [40], Mendia-Iztueta et al. [41], Andersson et al. [3], Carlsson et al. [34] |
Side-stepping G6, Low tuck G7, Plowing, Skidding, Downhill backwards herringbone HB, Downhill zigzagging, Sideways downhill movement are as in Table 1. |
2.1. Sixteen Techniques in Skate Skiing
2.1.1. Three Uphill Techniques Present in Both Skate Skiing and Classical Skiing
As our first technique, for extremely steep uphill terrain, the only possibility to get up the hill is using sideways uphill movement with parallel skis. The upper ski is moved sideways and upwards, and positioned into the snow, perpendicular or 90 degrees to the direction of movement, while the body tilts upwards. Thereafter the lower ski follows and is positioned next to and parallel with the upper ski. We estimate the degree of inclination as 45≤x≤90.
The second technique, uphill zigzagging, means skiing, placing one foot alternately before the other, just like walking but with parallel skis, moving to the left, then right, then left, etc., diagonally up a hillside. At a certain point, which may be after only a few meters or several hundred meters or kilometers depending on the terrain, sun, wind, need for variation, communication with one’s partner or group, etc., a turn of less than 180 degrees is made. The turn is usually made while facing uphill, with kicks, flips, ski removals etc., but can also be made while facing downhill and supporting with one’s poles. That is, the turn is usually clockwise if the previous walk was towards the left, and usually countered clockwise if the previous walk was towards the right. Subsequent clockwise and counter clockwise movement as preferred enables zigzagging up a hill. The switch from left to right (and from right to left) means coming to a full stop before turning the body less than 180 degrees, angling the skis in the air, and continuing in the opposite direction. The common clockwise turn while facing uphill is usually made in one of four ways, i.e. four different ways to complete the turn.4 The counter clockwise turn is usually made analogously in one of the same four ways. Various acrobatic and creative ways of turning are also possible.5
The third technique is the uphill herringbone technique HB, used in steep uphill terrain, and sometimes also in moderately steep uphill terrain. The skier faces uphill with the skis angling outwards, thus opening one’s legs so that the ski tips point outwards. The feet are somewhat apart to prevent the back parts of the skis to cross. Sufficient angling of the skis prevents the skier from sliding backwards. From this position, the skier positions each ski alternately onto the snow at a higher altitude along the hill, without gliding, while maintaining outward angling of the skis to prevent sliding backwards. The poles aid in the prevention of sliding backwards. As shown by Andersson et al. [11] in a study of 11 elite male skiers, the poling cycle consists of a poling phase during which the pole is planted, and a recovery phase during which the pole is swung. The leg movement consists of a thrust phase and a recovery phase. The right or left pole is planted before, in time, the ski on the opposite leg is planted (their Fig. 3c). At least one of the poles is in contact with the ground throughout the cycle. In contrast, the skis are not in contact with the ground during around 15% of the time for each cycle. For herringbone HB at high velocity, the lateral ski angulation is 29°, and the lateral pole angle when the pole is planted is 11°. Sandbakk et al. [10] found that a similar ski angulation is used in double dance G3 skate skiing (discussed below) in moderate uphill terrain (4.6°) at substantially higher velocity (4.7 m/s). Smith [12] found that the lateral pole angulation in the herringbone HB is similar to that of paddling G2 skate skiing (discussed below), and is not predominant in other classical skiing techniques. For the first three techniques we propose that the speed is less than 3 m/s.6
2.1.2. Six Techniques Unique to Skate Skiing
The next six techniques, i.e. gliding diagonal skate skiing G1, paddling G2, double dance G3, two skate G4, marathon skate, and combiskate G5 are used in successively easier terrain, transitioning from uphill to flat and downhill, and transitioning from high friction snow to low friction snow, accompanied with higher velocities.
Gliding diagonal skate skiing G1, sometimes used in steep uphill terrain, means herringbone HB with the addition of gliding. That is, as the ski is planted and weight is on the ski, it glides forwards before it comes to a full stop, after which the other ski is planted and glides forwards, alternately up the hill. We estimate speed usually less than 5 m/s.
Paddling G2 (also called V1, offset, gear 2), also a technique for skiing uphill, involves an asymmetrical and asynchronous double pole push in connection with every other leg push. The leg strides are nonsymmetrical. That is, the pole plant is asynchronous and combined with a skating stroke on one ski but not on the other ski [4]. The cycle starts with a pole plant on the strong side. The entire cycle consists of a single pole propulsion, with both poles, and two leg strides [13].
Double dance G3 (also called V2, one skate, 1-skate, gear 3), used on moderate inclines and level terrain, involves one double symmetrical pole plant and pole push together with every leg push, i.e. together with a skating stroke on each side [4, 13]. The double dance G3 skating technique is symmetrical. Using double dance G3 at inclinations of 2% or 8%, Sandbakk et al. [10] found that elite skiers at the 8% incline engaged in a longer propulsive phase, obtained higher work rates, applied more rapid cycle rates, and performed more work per cycle. On both inclines, increased speed followed more from cycle length than cycle rate. Longer cycle lengths followed from longer poling times, and longer pole and ski swing times. Double dance G3 is a high speed technique suitable for fast conditions on flat terrain, or to maintain momentum over short uphills. In moderate uphill 4–6° terrains skiers may use paddling G2 or double dance G3 [4].
Two skate G4 (also called V2A, V2 alternate, 2-skate, single dance, Gunde skate), used on relatively level terrain, is a symmetrical double pole push in connection with every other leg push, i.e. in connection with two leg strides. One cycle consists of one double pole propulsion and two leg strides [13]. Stoggl et al. [14] found that two skate G4 was 2.9±2.2% faster than double dance G3 in maximum speed sprints over 100 meters. Compared with double dance G3, they found that two skate G4 has longer cycle length, lower cycle rate, involves higher muscle activity, speed, and knee extension amplitudes, and higher peak foot forces, especially early during push-off.
For the techniques paddling G2, double dance G3, and two skate G4 Boulay et al. [13] and Kvamme et al. [4] found no speed differences for upward inclines up to ca 3°. For inclines above 4° Kvamme et al. [4] found paddling G2 to be more economical. Nilsson et al. [1] found that the phase durations and changes in duration are similar in paddling G2 and two skate G4. However, for two skate G4 the durations are symmetrical between the free and poling side. Paddling G2, double dance G3, and two skate G4 are characterized, at each speed level, by shorter duration of the thrust compared to the swing phase in the arm action. For the leg action, in contrast, the opposite is true.
Marathon skate (also called Siitonen-skate, Siitonen-step, finnstep) is another technique occasionally used on relatively level terrain, with narrow trails, tight racing conditions, when the groomed track is especially fast, tourist races, etc. It is the precursor to the modern skate skiing techniques or techniques that emerged 1984-1985.7 Its popularization is credited to Pauli Siitonen (born February 3, 1938). With good gliding, marathon skate may be applied in slight uphill terrain, and in slow or extremely slow snow conditions it may be applied downhill. One ski is kept straight and gliding, either locked within a track or on any surface, while the other ski is skate pushing, combined with one symmetrical double pole plant and push in connection with every leg push. The skate pushing ski is usually angled outwards 20-30 degrees. A larger angle is needed for slow snow conditions or for proceeding up a slight hill. A smaller angle is applied for faster snow conditions or for proceeding slightly downhill. The skate push should start with a push to the side from a one-foot forward position relative to the foot on the gliding ski which is not pushing, thus preventing the pushing leg from lagging behind the gliding ski. Too much time should not be spent on the skating leg which would cause the two legs to move apart (doing the split). Instead, just enough time should be spent on the pushing leg to enable pushing off, after which one glides on the gliding ski. After pushing off, one should relax to recover, and hence the name marathon skate, stand tall, and glide on the gliding ski, while the pushing leg swings forward getting ready for the subsequent push, and the poles are brought forward for the subsequent double pole push. Gervais and Wronko [8] found that the “Nordic Skate” roller skis more closely resembled the on-snow techniques than skating-specific roller skis, mainly due to discrepancies in the propulsion phase.
Combiskate G5 is skate skiing in downhill terrain, or in flat terrain typically after downhill, in a low position using only the legs, i.e. without poling [3]. It is used when high speed prevents effective pole utilization [1]. Leg movements on the left and right side of the body are symmetrical during thrust and swing. The thrust phase lasts longest, and decreases with speed from about 1.3 to 0.7 seconds. The swing phase lasts shorter, and decreases with speed from about
0.9 to 0.5 seconds.” For the previous techniques, i.e. paddling G2, double dance G3, two skate G4, and combiskate G5 and marathon skate we estimate speed less than 10 m/s.8
Millet et al. [18] compared the aerobic energy cost of paddling G2, double dance G3, two skate G4, and combiskate G5. First, they found lower energy cost and heart rate in paddling G2 than in double dance G3, likely due to paddling G2 utilizing the upper body more efficiently, with lower variation in the velocity of the centre of gravity. Second, aerobic energy cost was 5-9% higher in combiskate G5 than in paddling G2, double dance G3, and two skate G4, likely due to propulsive forces within a cycle lasting shorter in combiskate G5. Third, the correlation between performance and aerobic energy cost was significant for double dance G3, two skate G4, and combiskate G5, but not for paddling G2. Fourth, for paddling G2, double dance G3, two skate G4, and combiskate G5 the propulsive and total forces (mechanical efficiency) are higher in the upper than in the lower limbs.
2.1.3. Seven Techniques for Downhill and Turning Present in Both Skate Skiing and Classical Skiing
Side-stepping G6 is a step turning technique for curves in which leg work in the form of leg strokes are performed with or without poling [3]. It commences by lifting the inner ski or extending the outer leg for push-off [19]. The legwork ranges from stepping to active push-off, and involves an outward skating motion. The longitudinal axis of the skis coincides largely with the direction of movement, causing low ski–snow friction. Pushing occurs perpendicular to the direction of movement causing turning. Sandbakk et al. [20] found that more effective step turning is associated with lower energy dissipation towards the end of the turn. They also found that side-stepping G6 is more effective during all phases of a turn, causing higher velocities than skidding (described below). Side-stepping G6 certainly also causes higher velocity than plowing (described below) intended to effectively decrease the speed. Side-stepping G6 is classified as a propulsive technique when push-off is active, and as a technique for downhill velocity control/turning when merely stepping, and no active propulsion, is involved.
Low tuck G7 is downhill skiing in a low stance position without pole- and leg actions, i.e. no leg or pole push [3]. The knees are forward and the butt is towards the rear. The position partly resembles the squat position in weight lifting, but feet and legs are parallel, i.e. not tilted outwards. Skiers vary regarding how low they sit. A low stance decreases air resistance, but may be strenuous causing lactate buildup or otherwise restrict required circulation of blood and waste products in and through one’s legs. Thus skiers may choose a higher stance, or alter the height of the position. The poles are usually kept in place by keeping one’s arms close to one’s body, thus locking the poles to one’s body, pointed backwards, and preventing them from being dragged through the snow. Speed is usually above 2 m/s. Low tuck G7 is classified as both propulsive and for downhill velocity control/turning. Although low tuck G7 at speeds above 10 m/s prevents the skier from using legs and arms for locomotion, the skier adjusts his/her body and muscles optimally for optimal velocity control and maximum forward movement, thus not resisting forward movement.
We classify the last five techniques as follows. Plowing is used in steep downhill terrain, especially in narrow trails, through wooded areas, and in rough mountain terrain with cliffs. Plowing is also used downhill in terrain with twists and turns where one cannot look too far ahead. The skier slows down the speed by angling the skis in reverse V-formation with unloading movement, accompanied by lowering one’s body distinctly [19]. The legs are moved apart, the skis are angled inwards, and the front of the skis is kept close together without the skis crossing each other. By shifting the weight between the feet, and angling the skis differently, some direction change can be made. Gliding occurs along the skis’ inside edges. Turning towards the left or right occurs through changing one’s body position, adjusting the edge angle of the outer ski causing lateral ski-snow friction, and adjusting the weight on each ski. Speed is controlled by adjusting the edge angle and the reverse V-formation angle relative to the movement. This technique requires a certain sensitivity for the interaction between the skis and the ski surface, and is easier on even surfaces. Some powder snow on top of a hard surface makes the application of this technique easier.
Skidding is a technique used in steep downhill terrain with a curve where the skier in unloading movement and with a lowering of the body slows down the speed by keeping the skis together and parallel and slightly angled inwards towards the terrain [19]. That is, the parallel skis are directed at an angle relative to the direction of movement. Gliding occurs on the edge of the uphill ski to generate ski-snow friction. The back part of the skis is directed towards the outer side. Sandbakk et al. [20] found that skidding is more effective when deceleration is more pronounced early in the turn, while maintaining higher velocity thereafter.
For very steep downhill terrain where plowing, skidding, and step turning are impossible, the downhill backwards herringbone technique can be used.9 Alternatively, downhill zigzagging is the appropriate technique. It is similar to uphill zigzagging, but applied downhill. The difference is that instead of placing one foot alternately before the other uphill, the skier glides with parallel skis downhill, unless the conditions and abilities are such that walking is required. The skier comes to a full stop before turning the body less than 180 degrees, clockwise or counter clockwise as described above, and continuing in the opposite direction. The final technique, for extremely steep downhill terrain, where all the other techniques are impossible, is to move sideways downhill with parallel skis. Using empirics, Table 1 can be extended by accounting for additional factors such as the skier’s expertise, speed, wind, and snow conditions.
2.2. Fourteen Techniques in Classical Skiing
Table 2 classifies the 14 classical skiing techniques. The first three techniques and the last seven techniques are the same as those explained earlier for skate skiing. The four new techniques unique to classical skiing, labelled running diagonal stride RUN DS, diagonal stride DS, double poling kick DK, and double poling DP, replace gliding diagonal skate skiing G1, paddling G2, double dance G3, and two skate G4 for skate skiing.
2.2.1. Three Uphill Techniques Present in Both Skate Skiing and Classical Skiing
The first two techniques are sideways uphill movement and uphill zigzagging. The third is the uphill herringbone technique HB, used in steep uphill terrain or when skis lack sufficient grip for the diagonal stride. We define the herringbone HB to be equivalent in skate skiing and classical skiing, provided that gliding does not occur. Once gliding occurs in skate skiing combined with the herringbone HB, the technique is defined as gliding diagonal skate skiing G1, see section 2.1. The reason gliding incorporated into the herringbone HB is not permitted in classical skiing, as specified by FIS [21], is that the manner in which the skier’s legs are opened and the ski tips point outwards in the herringbone HB (see section 2.1) closely resembles the skier’s technique and bodily posture in ski skating. Hence intermediate techniques between the herringbone HB and the diagonal stride, i.e. any bodily posture involving herringbone HB combined with gliding, are also not permitted in classical skiing.
2.2.2. Four Techniques Unique to Classical Skiing
The running diagonal stride RUN DS is often used to accelerate and in sprint in short uphill slopes. It has no gliding phase and involves arms and legs moving in a coordinated pattern in opposition to each other to produce force mediated through the poles and skis to the ground. The movement resembles walking or running without skis. One arm pushes while the contralateral leg also pushes. One leg swings forward and comes to a stop. Thereafter a backward kick occurs involving quick downward and backward movement of the leg. This occurs for each leg alternately [5].
The diagonal stride DS is the major classical uphill technique. It is equivalent to the running diagonal stride RUN DS but with a gliding phase after kicking. The legs undergo a swing phase, a gliding phase, and a phase involving push-off flexion and extension, see [22] and [12]. The forward swing phase consists of a pendulum-like function of the swinging leg. It starts slowly and accelerates to a peak hip flexion angular velocity of 846°/s approximately 0.06 s before deceleration occurs and the ski is planted on the snow [22]. The peak angular velocity occurs when the swinging leg nears the bottom of its arc, immediately after the recovery leg passes the kicking leg. The ski plant does not cause the ski to come at a full stop as in the running diagonal stride RUN DS. Instead the ski starts gliding. Lindinger et al. [22] distinguish between “gliding low” and “gliding high”. “Gliding low” involves gradual weight transfer from the ski that kicks to the ski that glides. Increased weight transfer causes gradual transition to “gliding high” where the entire load is eventually shifted to the gliding ski. This load shift occurs while the body gets raised through leg joint extensions. As the gliding ski decelerates and comes to a stop, the next phase of push-off flexion and extension occurs. From a high hip position with extended hip and knee joints, it involves a rapid flexion and downward movement, similar to what occurs in double poling (discussed below). This transfers muscle force to the ground to enable good grip for the subsequent backward kick. Lindinger et al. [22] found that performance in diagonal roller skiing was linked to longer cycle length, greater impulse of force during a shorter push-off with larger flexion/extension ranges of movement in the leg joints, longer leg swing, and later peak pole force. Kehler et al. [23] found that the kinetic and gravitational
potential energy fluctuated out-of-phase during walking, and in-phase during running and the diagonal stride DS. However, the dissipation of kinetic energy as frictional heat during gliding in the diagonal stride DS could not be stored elastically in the tendons, as in running. Thus the diagonal stride DS resembles walking and running only superficially. Stoggl et al. [24] estimated maximum diagonal stride DS speed for 12 elite skiers on a treadmill with 1.5° upward incline finding 4.8±0.2 m/s. We thus estimate that the speed for the diagonal stride DS and the running diagonal stride RUN DS is commonly below 5 m/s. For skiing on flat ground, Hoffman [25] found the diagonal stride DS to be least economical, where 10-30% higher speed is obtained by the skate skiing techniques.
The double poling kick DK technique is used in slight uphill terrain with alternation of arm and leg propulsion through complex coordination of the arm swing and gliding phases. Compared with double poling (described below), it involves different coordination where the upper and lower limbs contribute differently. Whereas poling is similar to that of double poling, in double poling kick DK propulsion occurs through a left or a right leg kick [5]. Göpfert et al. [26] found that increased speed in double poling kick DK followed from increased cycle length and rate, and shortened durations for poling and leg push-off. Peak and average pole/leg forces increased, despite maintained impulses of force. During leg push-off and double poling, ranges of motion were maintained for hip/knee flexion and extension, and increased for elbow flexion and extension. Leg push-off varied substantially among elite skiers. Increases in arm swing time and average poling force correlated with increased cycle length. Changed double poling technique was the main method for speed adaptation.
The double poling DP technique is preferred by all athletes in flat terrain at a speed of 10 km/h≈2.78 m/s [5]. It is also commonly used in slight downhill terrain. It involves using the legs to position the body, but with propulsion solely from the poles. Both poles are moved symmetrically and synchronously. The propulsive action is enhanced by considerable trunk flexion. The legs’ involvement is minimal [5]. Holmberg et al. [27] observed that double poling DP has grown in importance as a main classical technique since the mid 1980s. They divided a double poling DP cycle into a poling phase and a recovery phase. One double poling DP cycle lasts from one pole ground contact to the next pole ground contact. They further found that the double poling DP velocity depends less on poling frequency, and more on pole force through activating the muscles in specific patterns and sequences. First the trunk and hip flexors are engaged. Second, the shoulder extensors and elbow extensor triceps brachii are engaged. Third, towards the end of the poling cycle, some specific complementary movements are added. The best skiers, adjusting their movement characteristics to the double poling DP velocity, focus on higher pole force applied during a shorter poling phase, higher flexion velocities, and smaller joint angles. At maximal double poling DP speed and diagonal stride DS speed, Stoggl et al. [24] found positive correlation between both repeatedly produced lactate values and skiing technical aspects on the one hand, and sprint performance on the other hand. They estimated maximum double poling DP speed on a treadmill with 1.5° upward incline finding 8.4±0.5 m/s. We estimate that speed for double poling DP and double poling kick DK is below 10 m/s, as also estimated for paddling G2, double dance G3, two skate G4, combiskate G5 and marathon skate in the previous section. Hoffman [25] suggested that increased use of double poling DP may be limited by greater anaerobic demands.
2.2.3. Seven Techniques for Downhill and Turning Present in Both Skate Skiing and Classical Skiing
Next comes side-stepping G6 which is the same step turning technique as in skate skiing. But, according to FIS regulations, in classical skiing side-stepping G6 can be used only to change directions, not to generate additional speed. This raises a possible grey zone regarding how to classify side-stepping G6 in classical skiing. We have chosen to classify it, uncontroversially, as a technique for downhill velocity control/turning when merely stepping, and no active propulsion, is involved. We have chosen, perhaps more controversially, to classify side-stepping G6 as a propulsive technique when push-off is active to maintain the current speed through a curve, without increasing the speed. The wording in future FIS regulations may perhaps settle this more clearly. Finally come low tuck G7, plowing, skidding, downhill backwards herringbone HB, downhill zigzagging, and sideways downhill movement, as in skate skiing.
3. RESULTS
Table 1 classifies six unique techniques identified and marked in bold for skate skiing. Table 2 classifies four unique techniques identified and marked in bold for classical skiing. Tables 1 and 2 classify 10 techniques for both skate skiing and classical skiing. This gives 6+4+10=20 techniques. To be exhaustive, we include ski mountaineering defined as skiing in extreme uphill and downhill terrains. Among the 10 techniques occurring in both skate skiing and classical skiing, two are for extreme uphill and three are for extreme downhill, marked in italics in Tables 1 and 2, and commonly not preferred in ski competition.
Skiers’ technique selections are determined by incline, skiing speed, whether a desire exists for propulsion or downhill velocity control/turning, frequency of time trained, the length of the uphill slopes, the combination of uphill, flat, and downhill, the total skiing distance, skiers’ expertise, recent training load, VO2max, exhaustion, motivation, nutritional preparation, equipment, weather including wind, temperature, humidity, etc. Tables 1 and 2 confine attention to incline, speed, and frequency of time trained, and refer to the literature for further reference. For example, Bilodeau et al. [28] found that the durations of the propulsive and gliding phases are longer for skate skiing than for the diagonal stride. In this paper we consider some factors. Other factors are either addressed in the literature or are topics for future research.
3.1. Technique Changes in Skate Skiing
Andersson et al. [3] found between 21 and 34 technique changes in a 1.45 km sprint skate skiing time trial. The fastest skiers used fewer technique changes. Confining attention to the most common techniques, i.e. paddling G2, double dance G3, two skate G4, combiskate G5, side-stepping G6, and low tuck G7 gives 30 possible technique changes for skate skiing.10 Some of these are common, and others never occur such as switches from paddling G2 to low tuck G7 or vice versa. Understanding the nature and logic of the 30 technique changes, when they shall be applied, how they shall be trained, etc., are useful in sports practice since lacking the required insight may lead competitors to perform technique changes more successfully. For example, if skiers lack experience about some technique changes, they may fail to perform them, and may choose wrong or suboptimal technique changes instead.
Shifting to the adjacent technique above or below is most common, but shifting two or three techniques up or down also occurs. A thorough analysis of technique changes means accounting for factors such as the terrain’s slope (uphill, flat, downhill), speed (and whether the skier accelerates, keeps the same speed, or decelerates), snow conditions, the skier’s expertise (professional or recreational) and exhaustion level, competition versus training, wind, and possibly whether the technique change occurs early in the race or close to the finish line.
Let us illustrate with technique changes in two typical terrains. Fig. (1) applies hypothetical reasoning to three techniques and two technique changes over a smooth hill. The skier starts with double dance G3 at the left in the moderate uphill terrain, and switches to two skate G4 as the terrain levels out towards the hill peak. This technique is maintained over the hill top, and the skier accelerates with combiskate G5 for the downhill. Fig. (2) illustrates based on hypothetical reasoning technique changes in more varied terrain. First, from the left, the skier maintains double dance G3 in the moderate uphill. Thereafter a switch occurs to paddling G2 as the uphill becomes steeper. This is in accordance with Kvamme et al. [4] who found that increasing uphill from 3°-8° makes double dance G3 increasingly costly compared to paddling G2. The transition at the top of the first hill towards downhill terrain is so abrupt that the skier may switch directly two techniques upwards, from paddling G2 to two skate G4 in skate skiing. This technique is maintained in the slight downhill, flat, and slight uphill terrain, until the skier again switches one technique downwards to double dance G3 as the uphill becomes steeper. As the new downhill approaches, which is less abrupt, the skier switches one technique upwards to two skate G4, i.e. skate skiing with symmetrical double pole push for every other leg push. Increased downhill gives combiskate G5, i.e. skate skiing without poling. Finally the skier switches to low tuck G7 in a low tuck position as the high speed prevents leg work and poling.
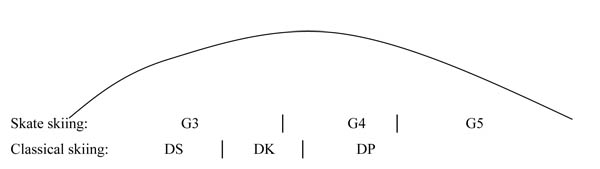
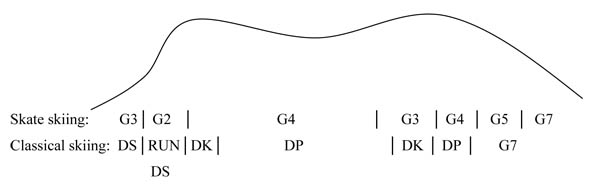
3.2. Technique Changes in Classical Skiing
Including uphill herringbone HB, and confining attention to the diagonal stride DS, double poling kick DK, double poling DP, side-stepping G6, and low tuck G7, also gives 30 possible technique changes.11 As in section 3.1 for skate skiing, some are common, and others rarely or never occur in practice, e.g. between herringbone HB and low tuck G7. For most technique systems shifting to the adjacent technique above or below is most common, due to commonly smooth changes in speed, incline, and various other factors. However, shifting two or three techniques up or down also occurs, especially when changes in speed, incline, etc. are abrupt. For example, starting from zero speed in a downhill position may allow skipping some techniques when accelerating through successively higher techniques. Alternatively, abrupt change from a high speed technique in downhill or flat terrain to uphill terrain, combined with slow snow conditions in the uphill terrain, may require skipping some techniques when changing to a suitable low speed technique. As in section 3.1, thoroughly analyzing technique changes means accounting for factors such as the terrain’s slope, speed, snow conditions, the skier’s expertise and exhaustion level, competition versus training, wind, and whether the skier approaches the finish line.
Three especially common techniques in classical skiing, from low to high, are the diagonal stride DS, double poling kick DK, and double poling DP. First, assume that skiers’ choice of technique depends on how they choose speed. The speed choice may be made freely during warmup, recuperation, for leisure, for tactical reasons, may be induced by exhaustion, or may be due to blockage by other skiers, narrow trails, or snow and weather conditions. As a function of speed, assuming a gentle uphill slope of 2°, skiers’ preferences are usually as follows [5]: At very low speeds, the diagonal stride DS is preferred. At higher speeds, skiers prefer the double poling kick DK or double poling DP. At speeds higher than 16 km/h≈4.44 m/s, all skiers prefer double poling DP.
Second, assume that skiers’ choice of technique depends on the incline. Pellegrini et al. [5] found that as the slope increases from 2° to 3°, skiers gradually switch from double poling DP to double poling kick DK. The added kick performed by the left or right leg in double poling kick DK becomes necessary with the increased incline. Double poling kick DK is kept until around 5°. At around 5°, a further change commonly occurs from double poling kick DK to diagonal stride DS. Skiers glide on each ski alternately after kicking with the leg attached to the other ski. At slopes steeper than 6°, all skiers apply the diagonal stride DS.
Björklund et al. [29] found for well-trained skiers that double poling DP at high intensity prior to the diagonal stride DS does not influence VO2, muscle activation or forces in the diagonal stride DS. Thus skiers may well utilize double poling DP to exhaustion well into uphill terrain, before switching down two techniques to the diagonal stride DS, assuming that the intermediate technique double poling kick DK is skipped.
Illustrating with technique changes in two typical terrains, Fig. (1) applies hypothetical reasoning to three techniques and two technique changes over a smooth hill. The skier starts with the diagonal stride DS at the left in the uphill terrain, and switches to double poling kick DK as the uphill incline decreases towards the hill peak. This technique is maintained until the hill top is reached, and for some skiers slightly beyond the hill top to enable acceleration. Thereafter the skier accelerates with double poling DP for the downhill. Fig. (2) illustrates based on hypothetical reasoning technique changes in more varied terrain. First, from the left, the skier maintains the diagonal stride DS in the moderate uphill. Thereafter a switch occurs to the running diagonal stride RUN DS as the uphill becomes so steep that gliding becomes impossible. As the top of the first hill is approached the uphill incline decreases so abruptly that the skier before the top is reached may switch directly two techniques upwards, from the running diagonal stride RUN DS to double poling kick DK. This technique is kept until the top is reached, and for some skiers somewhat beyond the top to enable acceleration, after which a switch to double poling DP occurs. Double poling DP is maintained in the slight downhill, flat, and slight uphill terrain, until the skier again switches one technique downwards to double poling kick DK as the uphill becomes steeper. If the switch to uphill had been more abrupt, the skier might have switched two techniques down, from double poling DP to diagonal stride DS, which is partly reminiscent of the switch from running to gallop in horse racing, which also causes lower speed. Around the top the skier again accelerates and switches upwards to double poling DP for the downhill, and eventually upwards to the low tuck G7 position as the high speed prevents effective poling.
4. DISCUSSION
This section discusses, sketches a research program for empirical analysis of technique changes in various terrain, and attempts to recommend a research program. The terrain can be such that only one technique is used throughout, e.g. based on the degree of inclination in Tables 1 and 2. The terrain usually varies so that more than one technique is needed. How these choices are made is crucial to determine in future research. It will become increasingly important for elite skiers to monitor their technique choices as improved measurement instruments become more easily available related to terrain and physiological characteristics. Future research should determine how elite skiers choose techniques through various terrains, and allocate training to the various techniques. In doing so, one should realize that six techniques are unique to skate skiing, four techniques are unique to classical skiing, 10 techniques occur in both skate skiing and classical skiing, and five of the latter occur in ski mountaineering.
More specifically, a research program involves determining how much time skiers with different expertise devote to each technique, and how they switch between techniques, depending on the terrain’s slope, snow conditions, friction, competition versus training, wind, where in a race one is located, the skier’s speed, acceleration, deceleration, and possibly the techniques and speeds chosen by the competing skiers, etc. A research program should account for the insight that a variety of such external factors influence technique choices. Additionally, technique choices are likely different on a treadmill and on snow, and skiers’ reporting of which techniques they use or believe they should use may differ from technique choices actually made in practice.
One systematic approach is to keep a constant slope of x degrees, where x is positive or negative, increase the speed gradually from 0 km/h to max speed and back to 0 km/h, and determine the chosen techniques at each speed, for skate skiing and classical skiing. The skier should be videotaped, wearing accelerometer, heart rate monitor, GPS for measuring speed and position, and a portable metabolic device to measure VO2. Using the data enables determining at which position a skier switches technique, and the speed at which he/she switches technique. Expected values and standard deviations for all measurements across all skiers are determined.
- Examples of alterations are that the skier can be instructed to
- choose his/her own techniques and his/her own pace with no instructions,
- keep a certain speed or reach a finish line within a specified or minimum time,
- accelerate and decelerate through certain speeds at preferred techniques,
- choose specified techniques in a specified succession, but with the ability to choose himself/herself where to switch technique depending on the speed and slope,
- be rested or exhausted before the test.
To support the analysis, interviewing professional and recreational skiers, the commonality of the various techniques should be determined, together with the percentage and total time used to train or exercise each technique.
The results in the results section reveal or suggest various hypotheses that may be tested. For example, for section 3.1 for skate skiing and section 3.2 for classical skiing, empirical analysis should count the number of times each of the 30 technique changes occurs depending on the various factors described. That will illustrate which technique changes are most vs least common. For example, we hypothesize that the technique change from double poling DP to the diagonal stride DS in section 3.2 for classical skiing, i.e. two techniques downwards, is successful only when the terrain suddenly changes to uphill, combined with substantial snow friction, which should be tested experimentally in future research. Skiers are commonly observed to make this technique change mistakenly when they become tired in their arms from using the double poling DP technique. We hypothesize that in most cases this technique change from double poling DP to the diagonal stride DS causes loss of momentum and thus low speed, while the opposite technique change from the diagonal stride DS to double poling DP causes increased speed, which should be tested experimentally.
The research program includes general race analysis of top-level races for a large number of competitors of both sexes at various levels, focusing on speed, techniques, slope, snow conditions, and the other factors mentioned above. That presupposes videos of all competitors throughout the race with a precision level sufficient to identify the techniques, a time indicator on the videos, a speed indicator (e.g. accelerometer on each competitor or speed deduction from the videos) linked to time and video, and the ability to measure the other factors either by inspecting the videos or the track or through interviews. The program requires several research partners. It enables attaching real numbers to the percentage of time each competitor uses each technique, which techniques are used at which inclines and which fitness levels, the numbers of changes between which techniques, etc. Compiling the numbers across multiple competitors, age groups, proficiency levels, and both sexes, enables determining expected values and variances, thus elucidating variability between competitors. Compiling the numbers for the same race track at different times enables assessing the dependence on other factors such as snow and weather conditions. Compiling the numbers for different race tracks, i.e. of different lengths, elevation changes, etc., enables further robustness checks. Similar types of (less complicated) competition analysis (scouting reports) have been made in several other sports. The research program will yield more robust results than the hypothetical numbers in Tables 1 and 2.
This paper has mentioned and to some extent discussed the challenge that technique changes depend not only on incline, speed, and various other factors, but also occur to enable the skier to utilize the upper and lower body muscles differently, some muscles resting while others are working. Muscles consist of different myofibrils aligned in parallel. The myofibrils consist of parallel/series contraction elements (myosin/actin) which in turn contain different types of contraction molecules or isoforms designated type I slow isoforms, type IIx fast isoforms with about 3-5 times higher contraction velocity, and type IIa isoforms with intermediate contraction velocity [30]. A muscle contains different fractions of the type I, IIa, and IIx isoforms, impacted by training. Insights such as these reveal various challenges related to letting some muscles rest while others operate.
Since these issues are relevant for technique changes, let us consider some relevant research. First, Fabre et al. [31] tested aerobic energy expenditure during skiathlon, i.e. 3 km skate skiing and 3 km classical skiing, in randomized order. They found that the transition between classical skiing and skate skiing alters skiing speed in skate skiing, and alters aerobic energy expenditure and neuromuscular function. Second, Grasaas et al. [32] tested changes in technique and efficiency where 12 elite male skiers exercised submaximally four minutes before and after double dance G3 to exhaustion on a 5% inclined roller-ski treadmill. They found that after high-intensity exercise, submaximal roller-ski skating was performed with less efficient technique and shorter cycle length, not explained by changes in ski forces or peak power in the upper and lower limbs. Third, Welde et al. [33] compared the energy cost of skate skiing and classical skiing compared with inclined running. For example, they found that the high-level junior females could not maintain intensity above their onset of blood lactate accumulation levels even in short races below 25 min, which suggests training around those accumulation levels. Fourth, Carlsson et al. [34] tested for the diagonal stride DS and double poling DP how the oxygen uptake at different intensities impacts sprint performance. They found that skiers with 1% higher oxygen uptake can expect 0.2% higher performance.
Apart from changes in technique are changes in style, e.g. from classical skiing to skate skiing, as in skiathlon studied by Fabre et al. [31], where skiers also change skis. The style change in skiathlon is in various regards comparable to style changes in triathlon which involves three different exercise styles or modes (swimming, cycling and running) including two transitions between the styles. Triathlon has received significant interest, as triathletes perceive difficulties in the transitions, especially from cycling to running, see Bentley et al. [35]. Style changes in cross country skiing have to the author’s knowledge not been analyzed. However, technique changes within classical skiing have been analyzed, as described by Pellegrini et al. [5] and Pellegrini et al. [36]. While style transitions in cross-country skiing are probably not as challenging as in triathlon, the extra energy costs and time lost while changing technique versus the unloading and variations of muscle load is interesting both from a physiological, biomechanical and motor control perspective and should be further investigated.
CONCLUSION
Techniques and technique changes are crucial for the outcome of a race in cross-country skiing. Efficient changes between techniques are essential to continuously choose the most economical and/or efficient technique, to increase or decrease speed over shorter periods of time when needed, and to move efficiently in between and around competitors. In this study we define, specify, classify, and illustrate six unique techniques for skate skiing, four unique techniques for classical skiing, and ten techniques present in both skate skiing and classical skiing. Five of the ten latter techniques are also present in ski mountaineering, which require different equipment such as climbing skins, special bindings, boots and poles, for extremely steep uphill and downhill terrains. How a skier chooses techniques depends on the terrain’s slope, snow conditions, friction, competition versus training, wind, where in a race one is located, the skier’s expertise, exhaustion level, speed, acceleration, deceleration, and the techniques and speeds chosen by the competing skiers. The skier’s speed in a certain terrain is influenced by the skier’s fitness, ski and snow characteristics. The skier’s individual characteristics, such as his/her ability to produce power with the upper and lower limbs, body height and mass, and the individual preferences based on earlier experience also influence the choice of technique. We illustrate the employment of techniques in various terrains and speeds, and sketch a research program for empirical analysis. Future research should discuss more thoroughly when, why, and how skiers change techniques in varying terrain, which plethora of factors impact technique changes, and what the implications are.
Among the many publications on cross-country skiing, this is the first to classify techniques systematically. Realizing that there are as many as six unique techniques for skate skiing, four unique techniques for classical skiing, and 10 remaining techniques for both skate skiing and classical skiing, is imperative. Among the 10 latter, the two extreme uphill techniques, i.e. sideways uphill movement and upward zigzagging, and the three extreme downhill techniques, i.e. downhill backwards herringbone HB, downhill zigzagging, and sideways downhill movement, occur in ski mountaineering and recreational skiing, and rarely or never in competitions. Thus a competitive skier needs to master 6+4+5=15 techniques. These are gliding diagonal skate skiing G1, paddling G2, double dance G3, two skate G4, combiskate G5 and marathon skate unique to skate skiing, the running diagonal stride RUN DS, diagonal stride DS, double poling kick DK, and double poling DP unique to classical skiing, and uphill herringbone HB, side-stepping G6, low tuck G7, ploughing, and skidding present in both skate skiing and classical skiing.
The actual number of 15 techniques raises a number of perspectives such as how to train these techniques, which techniques to master before which other techniques in one's maturation, and at which stages in one’s career, how to change between techniques (e.g. one or several techniques up or down), which techniques are advantaged by which bodily characteristics, which techniques to use and avoid in which situations, e.g. early in races, late in races, in long versus short races, and in crowded environments with body contact with other skiers where oneself or others may fall or get disqualified.
As cross-country skiing gets more competitive, realizing the presence of 15 techniques to be trained, the issue emerges which kinds of alternative training to conduct to master which techniques. For example, the increased prevalence of double poling DP [27] have increased some skiers’ focus on training crunches to enhance one’s stomach muscles. More generally, striking the right balance between cardiovascular training and focused strength training of particular muscles and muscle groups crucial for specific techniques should in the authors’ view get increased attention in future research.
Today’s available research has enhanced our understanding of some techniques and combinations of techniques. The exhaustive view of available techniques provided in this paper, combined with reviewing and illuminating the multiplicity of factors impacting choices of techniques and technique changes, enhance our perspective of cross-country skiing and illustrate research opportunities and future perspectives on techniques and changes between techniques.
ETHICS APPROVAL AND CONSENT TO PARTICIPATE
Not applicable.
HUMAN AND ANIMAL RIGHTS
No Animals/Humans were used for studies that are the base of this research.
CONSENT FOR PUBLICATION
Not applicable.
CONFLICT OF INTEREST
The author declares no conflict of interest, financial or otherwise.
ACKNOWLEDGEMENTS
I thank Øyvind Sandbakk and four anonymous referees and the Editor of this journal for useful comments.