All published articles of this journal are available on ScienceDirect.
The Added Effect of Respiratory Training on Stabilometric, Respiratory and Agility Outcomes Among Recreational Athletes: an Experimental Design
Abstract
Background
Diaphragm activation plays a crucial role in various function activities through the adequate core muscle setup. In order to bring a change in balance, agility, and respiratory outcomes in recreational athletes, the role of combined diaphragm and balance training is still under debate.
Purpose
The study aimed to investigate the added effect of respiratory training on balance training in recreational athletes.
Methods
Forty-two male recreational athletes were randomized into either the balance training group alone or the added respiratory training group.
Result
The result of the study revealed that the agility, balance, and respiratory outcomes were improved significantly (p < 0.05) in the combined respiratory and balance training group. However, in the balance training group seperately, the respiratory and agility outcomes did not improve significantly (p > 0.05), but the balance outcomes improved significantly (p < 0.05).
Conclusion
The study concludes that additional respiratory training can help enhance respiratory and agility outcomes compared to balance training alone.
1. INTRODUCTION
Respiratory muscle training (RMT) plays a crucial role in optimizing the overall performance of athletes through breathing retraining, inspiratory muscle training, etc [1]. The improved vital capacity and respiratory muscle endurance through RMT have a positive impact on fitness, cardiorespiratory parameters, quality of life, and exercise performance than the control [2]. Additionally, athletes with better respiratory control have reduced the work and energy cost of breathing and thus generate a greater adaptation [3, 4]. It enhances tissue metabolism and improves oxidative capacity, thereby reducing the acidification of body tissues following heavy exercises [5, 6].
Maintaining optimal respiratory function is essential for sports performance, particularly in endurance activities, where efficient airflow is critical for performance [7]. Assessing and addressing these disorders could lead to practical and advantageous improvements in athletes’ performance during training and competitions, thereby enhancing their overall performance [7].
Diaphragmatic excursion and inspiratory intercostal muscle training are two methods for improving the function of specific respiratory muscles. Reduced respiratory excursion, particularly diaphragm and intercostal muscle fatigue, has been demonstrated in studies to impair recreational athletes' capacity to gain performance in various track and field sports [8].
Athlete cardiopulmonary health is entirely dependent on aerobic endurance-based training. Loading the diaphragm and intercostal muscles during exercise training stimulates and adapts them in the same way as all other skeletal muscles in the body [9].
Inspiratory muscle fatigue causes a decrease in maximum inspiratory pressure, which results in the activation of the metaboreflex, which causes a decrease in the activation of autonomic modulation, which results in the redistribution of blood flow from peripheral muscles to respiratory muscles in response to increased cardiac overload, thereby decreasing blood flow to active limbs and inducing early fatigue [9]. According to studies, respiratory training is vital for avoiding respiratory muscle fatigue [8].
Recreational sports foster a calm and encouraging atmosphere that helps people socialize, form friendships, and enhance their general well-being [9]. It improves physiological and psychological well-being, promotes social and cultural cohesion, and involves competition at all levels. There are many add-on techniques that have been used in the sports field mainly to improve performance, manage anxiety, and enhance the recovery process after training and competitions [10]. Physical training among recreational athletes makes a substantial contribution to individual athletic ability and physical characteristics [10].
The importance of sport-specific performance in recreational athletes is underscored by the need for specialized training programs. Sport-specific fitness is a key component of performance and can be enhanced through the development of physical training. The vo2 max is positively related to maximal work performance. The vo2 max parameter is expected improve with athlete-specific training and changes in body composition. An optimal vo2 max parameter helps to comply with the metabolic requirements on the field [10].
Stabilometric assessment is a crucial tool in evaluating the postural stability of athletes, which can be affected by sport-related injuries and intrinsic factors [11]. This assessment can help identify discipline-specific imbalances [12] and predict the risk of subsequent sports injuries [13]. Athletes showing the worst center-of-pressure spread variables are more prone to sports injuries in the subsequent training period [12]. Uninjured female athletes demonstrate better postural stability on video-force plate analysis than their uninjured male counterparts [11].
Balance and motor control are fundamental to athletic success, influencing performance, injury prevention, and the ability to adapt to various challenges in sports. Improved balance contributes to better execution of skills, allowing athletes to perform movements more efficiently and effectively. Strong balance and motor control can help prevent injuries by enabling athletes to maintain stability during unexpected movements or changes in direction. This is particularly important in sports that involve rapid changes in pace or direction [14].
Researchers identified a link between balance, posture, and respiration. Despite its importance in sports performance, balance training is sometimes neglected among recreational players [15]. Balance is the foundation for stability, coordination, agility, and control, all of which are required in a variety of athletic activities. Balance training increases body control, response time, and injury prevention, allowing athletes to perform at their best while lowering the chance of falls and accidents [16].
Recreational athletes can greatly improve their overall athletic performance by incorporating balance training into their regimens [9]. Although research has been conducted on the effects of respiratory and balance training on sports performance, there is a dearth of studies that explicitly target recreational athletes. Most previous studies have concentrated on professional athletes or specific sports disciplines, neglecting the larger population of recreational participants [15, 16].
Moreover, the effect of respiratory training on balance outcomes was evaluated using field- or instrument-based tests [16, 17]. However, the inertia-based balance evaluation following the training is yet to be explored.
As a result, research into the effects of respiratory and balance training on recreational players is needed to provide significant insights that can assist this rising demographic. Additionally, to investigate the relationship between respiratory and balance training and athletic performance among recreational players. Thus, the study has attempted to investigate the function of respiratory and balance training in increasing athletic performance, specifically among recreational athletes.
2. MATERIAL AND METHOD
This experimental study with 42 subjects was carried out following approval from the institutional ethics committee (ABSMPT/20-2320). Before participating, all participants were given a consent form; there were no restrictions, and people could withdraw from the study by stating to the researcher. The block randomization technique using the chit method was used to divide the groups into either alone balance training or the combined respiratory and balanced training group.
- Male recreational athletes.
- Age between 18 to 25 years.
- Active training status- at least played least 1 hr/day and 2 days in a week.
- Athletes must be free from any Cognitive or psychoactive drug.
- Athletes that use normal turf shoes in their training and matches.
- History of any respiratory and cardiovascular disorders
- Any recent injury.
- Undergoing rehabilitation from the past 4 to 6 months.
- Any joint disorder or allergy.
2.1. Measurement Procedure
2.1.1. VO2 Max
Cardiorespiratory endurance was assessed using the Beep test, which measures Vo2max. The beep test is both reliable and valid. In this study, the researchers employed a 20-meter beep test, also known as the shuttle test or bleep test, to assess recreational athletes' aerobic fitness [17].
The 'Beep-Test' is a timed test performed to fatigue using multistage 20-meter shuttle runs for the prediction of VO2 max. The participants were asked to complete the 20-meter shuttle runs at a given speed as per the indications given by pre-recorded audio. The starting velocity is 8 km/h, and it increases by 0.5 km/h every 2 minutes. The test ends when a player either fails to complete the 20-meter distance two consecutive times within the allotted time or cannot complete the stage due to exhaustion [17].
2.1.2. Agility
The T-Test was used for agility assessment through forward, lateral, and backward movements [20].
The T-Test is a reliable and valid test to assess agility. It was conducted using the guidelines provided by the previous studies. The units of measurement were at a 10 ×10 m course. The participants were instructed to run forward to the center cone as quickly as possible using the “go” command. Then, the participants sidestepped to the right 5 m to the right cone and sidestepped to the left 10 m to the far left cone, followed by sidestepping back to the right to the center cone. The participants then ran or moved backward as quickly as possible to cross the finish line. Each trial's completion time was measured in seconds. A participant was deemed disqualified if they did not follow the course instructions, finished the course or reach the finish line, move any cones, did not maintain their feet and trunk pointed forward at all times, or repeatedly crossed their legs while sidestepping. A participant received a score of 0 if they were unable to successfully finish a trial.
2.1.3. Compared Proprioception and Stabilometric Assessment of Balance- was used for Measurement of Dynamic Postural Stability Indexes
The stabilometric assessment was performed by using the Prokin system (PK 252, TecnoBody, Bergamo, Italy) (Fig. 1). Techno-body is a monoaxial platform that consists of 3 strain gauges that were placed under a circular surface of 55 cm in diameter and has a 20 Hz sampling frequency. In addition, to balance on an unstable platform condition was too difficult for the athletes to maintain. Simultaneously, an adjustable belt that included a trunk sensor was placed on the sternum of all subjects to record the trunk angular deviation. The position of the trunk sensor was determined according to the software manual of the Prokin system. Every time before beginning the tests, the device was recalibrated. Each test was repeated 3 times, with a 3 minute rest in-between [21].
We used a stabilometric platform (Prokin 254 (Pro-Kin Software Stability), TecnoBody S.R.L., Dalmine, 24044 Bergamo, Italy). This device is a force platform with a flat and regular surface fixed to four force-transduction systems (Fig. 1). The platform sends the signals to a computer for offline analysis and for detecting the position of the centre of pressure (CoP). The CoP represents the point of application of forces concerning feet and ground. The CoP area is an index of the effectiveness of the tonic postural system in keeping the centre of gravity closer to the intermediate position of balance [21].
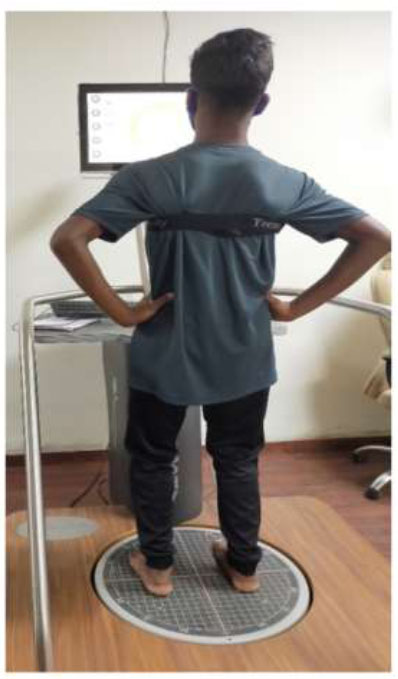
The stabilometric balance and proprioception assessment.
2.2. Procedure
All the participants in the combined respiratory and balanced training group received training for 30 minutes, followed by routine training. Similarly, the participants in the balance training group received only balance training for 30 minutes followed by routine training. The training frequency was thrice a week for six weeks. Each group of athletes warms up for 20 minutes before training, followed by a 10-minute cool-down time thereafter [12, 22].
2.3. Training Program
All baseline measurements were taken on the first day and reported for both groups on the second day. The balance training group alone merely performs a balance exercise, beginning with a double leg. Then, a single leg standing with eyes open and closed for one week, followed by star excursion training in the same advancing week, is performed (Fig. 2). On the second progressive week of training, wobble board training with double leg support is followed by single leg training with open eyes on the third week (Fig. 3). Wobble board balance with upper limb plyometrics, such as grabbing the ball in different directions followed by board balance and holding the weight from 2kgs to 5kgs, is included in the 4th to 6th week training regimens [23].
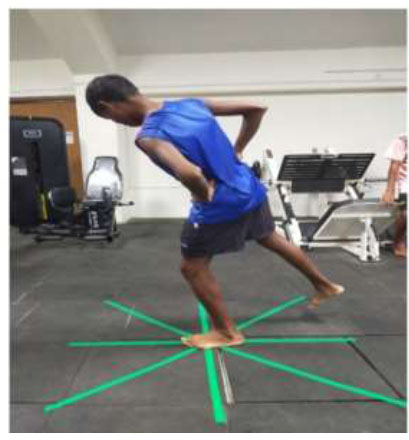
Balance training.
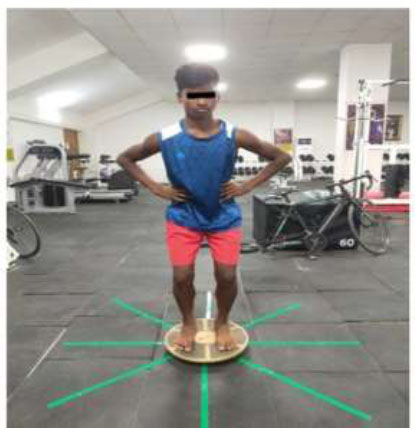
Wobble board training.
The balance training programs were scheduled 3 times per week for 6 weeks, a total of 18 sessions. Each session lasted for 30 minutes, and the number of exercises was 6 per session with 3 sets of 6 repetitions each.
The combined respiratory and balanced training group participants underwent both balancing and breathing exercises. Balance training adheres to the same guidelines as the balance training group, but the repletion and frequency were reduced.
The balance training was given 2 times a week for 6 weeks, a total of 12 sessions. Each session lasted for 20 minutes, and the number of exercises was 5 per session with 3 sets of 5 repetitions each.
Participants begin the respiratory training with diaphragmatic breathing exercises. Six breaths/minute and 4 to 5 sets with a 1-minute rest time followed by a breath hold beginning with a 1-minute increase up to 2 minutes with 5 repetitions over 2 weeks were performed. After 2 weeks, segmental breathing exercises were started with 3 sets on the third week, followed by 4 to 5 sets on the fourth and sixth weeks. Similarly, diaphragmatic breathing exercises and breath hold progress in 3 sets, 4 sets, and 5 sets every 2 weeks, 4 weeks, and 6 weeks were performed. Spirometer exercises were performed in addition to these workouts to increase volume progression [23-25].
2.4. Sample Size of Estimation
Sample size calculation was done by using the formula for comparative studies.
(Outcome measure – balance)
n = 2k SD2 / d2,
n = No. of Samples
k = Power
SD = Standard Deviation
D = MCID value
= 2*k (2.56)2/(3.32)2 ………… k = 10.5
d = MCID,
MCID = 1.96* √2 *SEM
SEM = 1.20
= 2* 10.5* 6.55/11
= 21* 0.59
= 12.39 … (Average of 13 per group)
Considering the dropout 42 sample size was considered for the study.
2.5. Statistical Analysis
SPSS version 26 was used for the data analysis. The Kolmogorov-Smirnov test was used for the normality analysis. The dependent t-test was used for time factor analysis, and the independent t-test was used for the group factor analysis. The level of significance was kept at 0.05.
3. RESULT
There was no difference in the demographic characteristics of the participants in both groups. The data were expressed using mean and standard deviation (Table 1). For the time factor analysis, the proprioception and the balance outcome had significantly improved in both the groups at the post-outcome assessment (p < 0.05). However, the VO2 max and the agility parameter did not improve significantly in the balance training group alone (p > 0.05) (Table 2). Similarly, for the group factor analysis, the mean difference between baseline and post-outcome assessment was considered for analysis, and the result revealed that all the outcomes were significantly improved (p < 0.05). However, the between-group difference was high in the balance outcome (d = 0.70), moderate in vo2 max (d = 0.46), and low in the proprioception outcomes (d = 0.18), and the changes were even smaller in the agility outcomes (0.02) (Table 2).
4. DISCUSSION
The current study aimed to investigate the balanced training vs. combined respiratory and balanced training on various sports performances among amateur athletes
The result of the study revealed that both the groups showed a significant improvement in the proprioception and balance parameters; however, the added respiratory training could improve only the VO2 max parameter and agility performances compared to the regular balance training group. Therefore, it can be confirmed that added respiratory training to the balance training was effective in the field-based assessment tests; however, the inertia-based stabilometric assessments were not as effective compared to balance training. Though both the groups significantly improved in some of the outcomes, the between-group differences were not clinically meaningful.
The evidence supports the positive impact of balance training on sports performance. Including balance exercises in athletes' training regimens can lead to improved stability, coordination, proprioception, injury prevention, and sport-specific performance [16, 26].
A study revealed that balance training can significantly improve dynamic balance and stability in female soccer players through control over the body’s center of gravity. Similarly, another study on basketball players showed that balance training and sports-specific balance training could result in better postural control and, thus, overall performance and better execution of their skills as well [11]. Moreover, balance training can also enhance agility and jump performances as well [12, 27, 28].
On investigating the role of respiratory muscle training, one study revealed that 4 weeks of inspiratory muscle training could improve pulmonary function as well as sprint performance. Similarly, another study with progressive inspiratory muscle loading was able to obtain a significant change in respiratory function and cardiovascular endurance. Likewise, in our study the added respiratory muscle training was also able to bring a positive change in the agility, balance, and proprioception outcomes [24, 25, 29, 30].
It has already been proven that respiratory training has improved respiratory function, sprint time, and overall cardiovascular endurance through arteriovenous difference and lung function [29, 30].
However, in our study, due to a lack of improved motor control and the inadequate sensory structural feedback inputs following the respiratory training, were not able to develop significant changes in the balance and proprioception outcomes on the stabilometer platform. In order to improve the balance and proprioceptive outcomes using a inertia-based platform, requires more involvement of the nervous system on how well an individual identifies a stimulus and how quickly he responds to it. All these activity requires proper planning and execution where the brain helps with planning and the body parts execute accordingly. Nevertheless, the respiratory training could not bring all these changes similar to the required sensory-motor coordination, and thus, there was no effect on inertia-based outcomes.
However, the field-based outcomes of the agility and the beep test for Vo2 max outcomes in the added respiratory training group could improve significantly compared to the balance training group alone. For this finding, the authors could hypothesize that the increased heart rate during the inspiration increased the oxygen demand, and the change in both the length and strength of the respiratory muscles through diaphragm excursion following the diaphragmatic breathing could lead to greater oxygen demand. Similarly, the increased intra-segmental mobility also might have the potential factor for the improved vo2 max parameter in the respiratory training group compared to the balance training group alone [29].
Additionally, the improved respiratory muscle endurance enables one to maintain a high level of performance during quick and repetitive tasks. Similarly, the effectual removal of carbon dioxide and other waste products promotes faster recovery and, thus the, intense agility performance. Meanwhile, the better mental focus achieved through the enhanced respiratory capacities was able to be mentally sharp, and that can induce faster decision-making and precise movements [30].
The study had several limitations first, there was no control group that would have shown the difference between usual care and the experiments. Second, the athletes were heterogenic, such as belonging to different sports groups, not to a specific sport category, and this would potentially confound the result with different types of training and possibly different baseline characteristics. The protocols were given in adherence to their routine training, so the findings cannot be generalizable to the true treatment effect. The role of guideline-based or impairment-based respiratory training in sports is still under debate. However, the effect is already established in track and field athletes, the findings are still unclear on different sports, and thus, future studies need to be performed on highlighting the effect of impairment-specific protocol rather than being generalized to every athlete.
Variables | Combined | Balance Alone |
---|---|---|
Number | 20 | 20 |
Age | 16.4 (2.3) | 16.0 (2.5) |
Height (m) | 1.56 (.98) | 1.48 (1.2) |
Weight (kg) | 62 (8.9) | 65 (5.3) |
BMI (kg/m2) | 20.3 (2.5) | 21.4 (1.9) |
Outcomes | - | Combined | Balance | Between Group | ||||
---|---|---|---|---|---|---|---|---|
Time |
Mean (SD) |
P- value | Mean (SD) | P- value | Group Difference (Mean) | Effect Size (Cohen's d) | P-value | |
VO2 max | Pre | 43.77 (±3.67) |
0.05 | 44.22 (±5.3) |
0.53 | 0.84 | 0.46 | < 0.05 |
Post | 47.53 (±3.75) |
45.39 (±5.3) |
||||||
Agility T-test | Pre | 12.51 (±0.96) |
0.001 | 11.94 (±0.98) |
0.13 | 0.37 | 0.02 | < 0.05 |
Post | 11.77 (±0.96) |
11.6 (±0.71) |
||||||
Proprioception | Pre | 105.4 (±20.12) |
0.00 | 122.18 (±24.06) | 0.00 | 7.6 | 0.18 | < 0.05 |
Post | 34.46 (±4.7) |
32.97 (±10.52) |
||||||
Balance both feet | Pre | 0.95 (±0.54) |
0.012 | 1.43 (±0.61) |
0.02 | 0.41 | 0.70 | < 0.05 |
Post | 0.58 (±0.21) |
0.93 (±0.39) |
CONCLUSION
The study concludes that to improve the cardiorespiratory outcomes in recreational athletes, supplementary respiratory training can be added to the regular training program to have better respiratory muscle strength and, thus, overall performance. Respiratory training can be helpful for improving the field-based outcomes like agility; however, any inertia or platform-based outcomes may not benefit from the addition of supplementary respiratory training. Though some of the outcomes did not produce meaningful changes in the respiratory training, the individual responses may vary, and the extent of improvement may purely depend on some factors such as the athlete's baseline fitness level, training regimen, and sport-specific demands.
HIGHLIGHTS
• The study highlighted the added effect of respiratory training on both field-based and platform-based tasks among recreational athletes.
• This is the first study to explore the role of respiratory and balance training on inertia-based outcomes among recreational athletes.
• The study reported that adding respiratory training to the balance program has no significant effect on inertia-based balance outcomes (p > 0.05). However, the field-based beep test and T-test were significantly improved (p < 0.05).
AUTHOR’S CONTRIBUTIONS
The authors confirm their contribution to the paper as follows: conceptualization, data collection, writing: KB; Contribution – conceptualization, writing, manuscript preparation: PV. Contribution – statistical analysis, manuscript preparation: DP. All authors reviewed the results and approved the final version of the manuscript.
LIST OF ABBREVIATIONS
RMT | = Respiratory muscle training |
CoP | = Center of pressure |
ETHICS APPROVAL AND CONSENT TO PARTICIPATE
The study was approved by the institutional ethics committee of Abhinav Bindra Sports Medicine and Research Institute, India (ABSMPT/20-2320)
HUMAN AND ANIMAL RIGHTS
All procedures performed in studies involving human participants were in accordance with the ethical standards of institutional and/or research committee and with the 1975 Declaration of Helsinki, as revised in 2013.