All published articles of this journal are available on ScienceDirect.
Ascorbic Acid Supplementation and Immune Response in Healthy Women during High-intensity Exercise
Abstract
Introduction
the benefits of consuming extra Ascorbic Acid (AA) while exercising are not well understood.
Aim
This study aims to explore the effects of AA supplementation on oxidative stress, the immunological response, and inflammation in healthy women after a single bout of high-intensity exercise.
Materials and Methods
In a crossover design, 20 sedentary women (aged 18-22) underwent 30 minutes of vigorous cycling. They were divided into two groups: one receiving daily 1,000 mg ascorbic acid supplementation (1,000AA) and the other without supplementation (0AA). This regimen was maintained for one week. Blood samples obtained pre-exercise, immediately post-exercise, and 24 hours post-exercise underwent analysis for oxidative stress, inflammatory markers, CD4+ and CD8+ lymphocytes, and neutrophil phagocytic activity.
Results
An AA supplement significantly increased plasma concentrations of AA (p<0.05) and reduced post-exercise plasma MDA levels (p<0.05) but did not affect creatine kinase activity. White blood cells, CD8+ T cells, and IL-6 increased significantly after exercise but remained unchanged in the 1,000AA group compared to the 0AA group, while the neutrophil count increased (p<0.05) after exercise with no change in phagocytic function. A slight drop in phagocytic function was observed 24 hours after exercise in the 1,000AA group. Exercise and AA supplements had no effect on CD4+ T cells.
Conclusion
a single session of high-intensity exercise caused oxidative stress, muscle injury, and inflammation, as well as a transient increase in CD8+ T cells. A short administration of AA attenuated the exercise-induced oxidative stress and reduced inflammation by limiting the increase in IL-6 and CD8+ T cells.
1. INTRODUCTION
Understanding the body's responses and adaptations to physical activity is crucial for enhancing endurance. Adequate recovery, along with proper nutrition or supplementation, is essential to prevent injuries and ensure continual performance improvement [1]. A single bout of exercise can set off multiple processes that result in the generation of free radicals, such as Reactive Oxygen Species (ROS) and Reactive Nitrogen Species (RNS) [2]. The neutralization of these free radicals occurs through the action of a complex network of enzymes, including catalase, Superoxide Dismutase (SOD), glutathione peroxidase, and various non-enzymatic antioxidants, including vitamins A, E, and C, glutathione, ubiquinone, and flavonoids, to maintain a healthy balance of free radicals in the body. Excessive free radicals can damage cells and result in a variety of health problems. It has been thought that exercise promotes changes in the immune-neuroendocrine axis and blood levels of metabolites that directly affect how immune cells work [3, 4]. Exercise-induced tissue damage may activate inflammatory cells such as neutrophils, which then cause nicotinamide adenine dinucleotide phosphate (NADPH) oxidase to produce free radicals. These injuries to skeletal muscle, connective, and bone tissue are considered temporary and reversible because they cause an acute inflammatory reaction that is triggered, in part, by neutrophils and macrophages, which are responsible for removing waste products, repairing, and developing injured tissues [5]. Athletes frequently take antioxidant supplements to prevent muscle deterioration and fatigue as well as to improve performance, owing to the role that antioxidants play in scavenging free radicals [6]. Conversely, vitamin C (ascorbic acid; AA) has been observed to suppress cell signaling associated with training, including ROS generated during exercise [7, 8]. A previous study of 30 healthy men aged 18-35 with plasma vitamin C concentrations less than 45 mol/L found that taking vitamin C prophylactically had some benefits [9]. However, the potential advantages of taking vitamin C supplements before exercising remain largely unknown within the current scientific discourse.
The antioxidant properties of vitamin C play a crucial role in protecting cellular organelles against oxidative damage [10]. A deficiency in vitamin C leads to compromised immunity and heightened susceptibility to infections [11]. Our previous study showed that taking an ascorbic acid supplement for three months prior to moderate-intensity exercise was associated with intermittent and slight declines in the blood enzyme activities of catalase, glutathione peroxidase, and superoxide dismutase [12]. Pre-cycling supplementation with 1,000 mg of ascorbic acid enhances antioxidant capacity without preventing muscle damage during moderate effort [13]. Similarly, we demonstrated that consuming ascorbic acid seven days before a single moderate-intensity exercise session did not enhance phagocytic activity or antioxidant status but inhibited the exercise-induced increase in CD4+ T cells [14]. It is unfortunate that there are few studies in the literature that delve into the effects of ascorbic acid supplementation and exercise on immune response [14-16], particularly among sedentary healthy women. Currently, there is limited scientific evidence supporting the efficacy of high doses of vitamin C supplementation in enhancing immune function among healthy individuals [17].
The impact of exercise on immune markers depends on factors such as exercise intensity, especially in high-performance sports, as well as training duration, load, and recovery time [18]. Despite extensive research on exercise and immune function, there remains a notable gap in our understanding regarding how exercise specifically affects the response of immune cells in sedentary adults who are using ascorbic acid supplements. Therefore, our investigation aimed to determine if 1 week of ascorbic acid supplementation might modify exercise-induced alte- rations in oxidative stress markers, the immunological response, and inflammation in healthy women following a single bout of high-intensity exercise.
2. MATERIALS AND METHODS
2.1. Participants and Design
Twenty untrained female adults aged 18-22, participated in the study. Prior to providing written consent, all participants were informed of the purpose and conditions of the study. The study was conducted in accordance with the Declaration of Helsinki, and the protocol was approved by the Ethics Research Committee from the Faculty of Associated Medical Sciences, Chiang Mai University (AMSEC-62EX-040). An interview was used to recruit volunteers. Excluding smokers and individuals on regular medications, participants refrained from alcohol use during the experiment while maintaining their usual physical activity and dietary habits. A randomized, cross-over study was conducted (Fig. 1). Participants were sedentary for at least one year without a regular exercise program and had no history of liver, renal, metabolic, cardiovascular, or pulmonary diseases or orthopedic limitations in exercise tests. Randomly divided into two groups, the 0AA group (n = 10) did not receive ascorbic acid before the first exercise bout, while the 1,000AA group (n = 10) received 1,000 mg of ascorbic acid daily for 7 days from the Government Pharmaceutical Organization in Bangkok, Thailand. Participants were instructed to take an AA supplement every morning and keep this routine going through the day of the last blood draw, which was done 24 hours after the exercise. Before exercise, measurements of height and body weight were taken, and the Body Mass Index (BMI) was calculated. Participants in the study fasted for 2-4 hours before completing a 30-minute indoor stationary cycling workout at 75-85% of their maximal heart rate. The equation (220 - age in years) predicted participants' age-based maximum heart rate. Before the second cycle, following a one-week “washout period,” each participant received the opposite treatment prior to exercise. Heart rate was continuously monitored using a pulse oximeter (P030, Beurer GmnH, Ulm, Germany) during exercise to estimate energy consumption and maintain exercise intensity. Participants were allowed to drink water according to their thirst during and after exercise. Blood samples were collected in the morning after fasting for 2-4 hours before exercise, immediately post-exercise, and 24 hours post-exercise. Samples were stored in ethylenediaminetetraacetic acid (EDTA) and heparinized tubes at 4°C. Plasma obtained from heparinized blood samples was frozen at -20°C for subsequent analysis.

Illustration of the design and analysis of a crossover trial. Female participants took 2 capsules daily for 7 days, containing either 0 mg (0AA) or 1,000 mg of ascorbic acid (1,000AA). The indoor exercise involved a 30-minute session on a stationary bicycle at 75-85% of the maximum heart rate. A one-week washout period separated the two exercise bouts. Arrows indicate when blood samples were taken: before exercise (1st), immediately after exercise (2nd), and 24 hours post-exercise (3rd).
2.2. Determination of Routine Blood Chemistry Analysis, Non-Esterified Fatty Acid (NEFA), Hydroxybutyrate, and Total Creatine Kinase (total CK)
Plasma glucose (measured by glucose hexokinase), uric acid (using uricase), alanine aminotransferase (ALT, assessed without pyridoxal phosphate according to the International Federation of Clinical Chemistry, IFCC), creatinine (via an enzymatic method), total cholesterol (determined with cholesterol oxidase), triglyceride (analyzed using the glycerol-3-phosphate-peroxidase method), NEFA, hydroxybutyrate, and total CK (measured following IFCC guidelines) were quantified utilizing reagents and an automated clinical chemistry analyzer (BioSystems BA400, manufactured in Barcelona, Spain). This analysis was conducted in conjunction with the use of commercially available control and calibration kits (BioSystems Reagents and Instruments, Spain), ensuring the accuracy and reliability of the measurements.
2.3. Determination of Plasma TBARS
Malondialdehyde (MDA) levels in plasma were obtained from reactive components of thiobarbituric acid (TBA) [19]. TBARS is a widely used measurement for lipid peroxidation products, often employed alongside more specific tests like high-performance liquid chromato- graphy. In the TBARS assay, one molecule of MDA reacts with two molecules of TBA, forming a pink pigment with a peak absorption at 532 nm. Results were evaluated using a standard solution of 1,1,3,3-tetramethoxypropane (Sigma-Aldrich, St. Louis, MO, USA) and expressed in μmol/L.
2.4. Determination of Total Antioxidant Status (TAS), SOD, Ascorbic Acid and Interleukin-6 (IL-6)
Following the manufacturer's guidelines, TAS and SOD quantification was conducted using 2,2’-azino-bis(3-ethylbenzothiazoline-6-sulfonic acid) (ABTS+) radical formation and RANSOD Kit, respectively (Randox Laboratories, Ltd., Crumlin, UK). The test underwent analysis using an automated clinical chemistry analyzer, with control and calibration procedures carried out using the BioSystems BA400. Plasma ascorbic acid was determined using an ascorbic assay kit (Sigma-Aldrich, St. Louis, MO, USA) according to manufacturer instructions. Plasma IL-6 levels were measured using commercially available enzyme-linked immunosorbent assays (ELISA, BioLegend, San Diego, CA, USA).
2.5. Determination of Hematological Parameters
A Sysmex KX-21 three-part differential auto analyzer (Sysmex Corporation, Japan) was utilized for a Complete Blood Count (CBC) analysis on the same day the blood was collected into an EDTA tube.
2.6. Assessment of Peripheral Neutrophil Functions
To determine the phagocytic index, the blood from heparinized tubes was mixed with Candida albicans (DMST 21424, Department of Medical Sciences Thailand, generously donated by Dr. Khajornsak Tragoolpua). Candida albicans was cultured in Sabouraud's broth with 2% dextrose for 24 to 48 hours at 37 °C to obtain yeast-phase organisms. Adjusting the C. albicans suspension in normal saline solution (NSS) to 2.8×108 CFU/mL using a spectrophotometer (Shimadzu UVmini-1240, Japan) set at 620 nm, a neutrophil-to-yeast ratio of 1:1 was used to combine yeast cells with whole blood. The mixture was incubated at 37°C for one hour with periodic agitation every 10 minutes. Subsequently, the assembly underwent centrifugation at 1,500 x g for 5 minutes. After discarding an equal amount of supernatant as the yeast solution, the remaining whole blood was used to prepare air-dried smears stained with Giemsa. Counting 100 neutrophils on each slide, positive cells were defined as neutrophils that consumed Candida. Phagocytic activity was calculated as the ratio of positive cells to 100 neutrophils. The phagocytic index was determined by multiplying total Candida/positive neutrophils by phagocytic activity.
2.7. Immunophenotyping of Peripheral Blood Lymphocytes
EDTA blood was employed for immunophenotyping peripheral blood lymphocytes using a Beckman Coulter Cytomics FC 500 flow cytometer (Beckman Coulter Inc., USA) and three monoclonal antibody combinations. These combinations comprised CYTO-STAT triCHROME CD45-FITC/CD4-RD1/CD3-PC5 and CYTO-STAT triCHROME CD45-FITC/CD8-RD1/CD3-PC5 (Beckman Coulter, Inc., USA) for identifying CD4+ and CD8+ T cells, respectively. In a brief procedure, 100 µL of whole blood was incubated with 5 µL of each antibody combination in separate tubes in the dark at room temperature for 15 minutes. After lysing red blood cells with 500 mL of lysing solution (OptiLyse C lysis solution, Beckman Coulter Ireland Inc.), tubes were vortexed, incubated in the dark at room temperature for 15 minutes, and then centrifuged at 1,250 x g for 5 minutes. The pellet was resuspended in 1 mL of 1 percent paraformaldehyde in phosphate-buffered saline (PBS) after washing three times with 2 mL of PBS, followed by flow cytometer analysis. The gating technique, based on CD45, was used to detect all White Blood Cells (WBCs), followed by a CD3/CD4 histogram to calculate the percentage of CD4+ T cells in about 2,500 lymphocytes. The absolute CD4+ count, expressed as the number of CD4 cells per microliter of blood, was obtained by multiplying the percentage of CD4+ cells by the absolute lymphocyte count. The same strategy and calculations were applied for CD8+ counting in a second tube.
2.8. Statistical Analysis
By the end of the study, each cohort had n = 20 participants. Results were presented as a mean ± SE. Standard statistical software SPSS 18.0 was used to process the data (SPSS Software, Thailand). Two-way repeated measures analysis of variance was utilized, and differences between exercise (pre-, post-, and 24 hours post-exercise) and supplements (0AA and 1,000AA) were then detected using an LSD post-hoc analysis with statistical significance of p<0.05.
3. RESULTS
3.1. Participant Characteristics
Table 1 displays the characteristics of the study participants. All study participants with normal levels of blood cholesterol (191±10 mg/dL), triglycerides (72±5 mg/dL), and uric acid (4.5±0.2 mg/dL) demonstrated normal kidney and liver function, as indicated by tests of blood creatinine (0.98±0.02 mg/dL) and blood ALT (7±0.6 U/L). The Body Mass Index (BMI), calculated from a person's weight and height, was within the normal range. Additionally, there were no significant differences in terms of average heart rate during activity, percentage of heart rate during exercise, or caloric expenditure between the 1,000AA and 0AA groups.
3.2. Changes in Levels of Oxidative Stress Markers, Muscle Damage Markers, Glucose, Ascorbic Acid, NEFA, Hydroxybutyrate, and Inflammatory Marker
Table 2 illustrates the immediate impact of high-intensity exercise on oxidative stress indicators, including TAS, MDA, and SOD levels. Plasma TAS and SOD levels exhibited no significant changes in either the 1,000AA or 0AA groups. Notably, the MDA level showed a significant increase (p<0.05) immediately after exercise in the 0AA group, contrasting with the 1,000AA group, where such an increase was not observed. Furthermore, MDA levels returned to baseline 24 hours post-exercise in both groups. The immediate post-exercise MDA levels in the 0AA group were significantly higher (p<0.05) than those in the 1,000AA group. Following exercise, both the 1,000AA and 0AA groups displayed an increase in plasma total CK (p<0.05). Blood glucose levels remained within the reference range in both the 0AA and 1,000AA groups; however, the glucose level in the 1,000AA cohort was significantly higher (p<0.05) 24 hours after exercise compared to immediately after exercise. Plasma levels of NEFA and hydroxybutyrate did not change significantly between the 1,000AA and 0AA groups. Participants who consumed 1,000AA had significantly higher levels of plasma AA before and after exercise than those who consumed 0AA (p< 0.05). Furthermore, 24 hours after exercise, plasma levels of AA were significantly higher in both the 1,000AA and 0AA groups (p<0.05) compared to pre-exercise values. The baseline AA levels showed an increase from 3.17±0.54 mg/L to 3.72±0.62 mg/L after seven days of supplementation, providing evidence of participants' compliance with the experimental protocol. Immediately after exercise, IL-6 concentrations in 0AA increased significantly (p<0.05) before returning to baseline 24 hours later. However, the level of IL-6 did not change significantly in the 1,000AA group.
3.3. Changes in the Population of White Blood Cells
The white blood cell count rose (p<0.05) immediately after exercise in the 0AA group before returning to baseline 24 hours later, while in the 1,000AA group there were no significant changes (Table 3). After exercise, the neutrophil counts of both the 1,000AA and 0AA groups were significantly higher (p<0.05) than they were prior to exercise. However, after 24 hours post-exercise, the absolute counts of monocytes, basophils, and eosinophils decreased significantly in the 0AA group but remained stable in the 1,000AA group. There was no significant difference in lymphocytes between the two groups.
Parameter | 0AA | 1,000AA |
---|---|---|
Age, (years) | 21.2±0.2 (18-22) | |
Ethnicity/race, (n) | Southeast Asian (Thai) 20 | |
Height, (m) | 1.60±0.89 (1.53-1.67) | |
Weight, (kg) | 54.2±2.4 | 54.4±2.4 |
BMI | 21.2±0.9 | 21.3±0.9 |
Average heart rate (beat/min) | 150±1.7 | 145±2.2 |
% of heart rate | 79±0.8 | 77±1.1 |
Calories, (kcal) | 203±4.2 | 200±3.3 |
Note: Values are means ± SE; n=20 per cohort. 0AA, 0 mg of ascorbic acid; 1,000AA, 1,000 mg of ascorbic acid; m, meter; kg, kilogram; BMI, body mass index; min, minute; km, kilometer; kcal, kilocalories.
Test | 0AA | 1,000AA | ||||
---|---|---|---|---|---|---|
Pre-exercise | Post-exercise | 24 h post-exercise | Pre-exercise | Post-exercise | 24 h post-exercise | |
TAS, mM ascorbic acid equivalent | 2.01±0.02 | 1.93±0.03 | 2.01±0.05 | 2.01±0.02 | 1.89±0.03 | 2.01±0.05 |
Plasma TBARS, (μmol/L) | 7.2±0.5 | 8.9±0.8 * | 7.2±0.8 ** | 7.4±0.7 | 7.1±0.8 $ | 6.8±0.8 |
Plasma SOD, (U/mL) | 1.15±0.13 | 1.19±0.12 | 1.07±0.11 | 1.00±0.12 | 1.10±0.12 | 1.00±0.12 |
Total CK, (U/L) | 61±4.9 | 79±3.7 * | 83±6.9 | 61±5.6 | 70±6.5 * | 65±5.3 |
Glucose, (mg/dL) | 99±3.4 | 91±2.9 | 98±2.7 | 96±2.5 | 89±3.1 | 102±3.9 ** |
NEFA, (mmol/L) | 1.17±0.07 | 1.11±0.07 | 1.01±0.07 | 1.11±0.07 | 1.05±0.06 | 1.07±0.08 |
Hydroxybutyrate, (mmol/L) | 0.20±0.02 | 0.19±0.02 | 0.20±0.02 | 0.20±0.02 | 0.20±0.02 | 0.22±0.02 |
Ascorbic acid, (mg/L) | 3.17±0.54 | 3.17±0.53 | 4.12±0.55 * | 3.72±0.62 $ | 4.23±0.53$ | 4.53±0.89 * |
IL-6, (ng/L) | 4.51±0.63 | 5.31±0.57 | 4.70±0.70 | 4.19±0.52 | 4.62±0.69 | 4.58±0.58 |
Note: The exercise involved a 30-minute session on a stationary bicycle indoors, maintaining an intensity at 75-85% of the maximum heart rate. The values are means ± SE; n=20 per cohort. The p-values indicate the results of an LSD post-hoc analysis. *, p<0.05 compared with the pre-exercise value (within the group). **, p<0.05 compared with the post-exercise value (within the group). $, p<0.05 compared with the same time point (in between groups). 0AA, 0 mg of ascorbic acid; 1,000AA, 1,000 mg of ascorbic acid; TAS, total antioxidant status; TBARS, thiobarbituric acid reactive substances; SOD, superoxide dismutase; U/mL, unit per milliliter; Total CK, total creatine kinase; U/L, unit per liter; mg/dL, milligram per deciliter; mg/L, milligram per liter; IL-6, interleukin-6; ng/L, nanogram per liter; NEFA, non-esterified fatty acid; mmol/L, millimole per liter.
Parameter | 0AA | 1,000AA | ||||
---|---|---|---|---|---|---|
Pre-exercise | Post-exercise | 24 h Post-exercise | Pre-exercise | Post-exercise | 24 h Post-exercise | |
WBC (103 cell/µL) | 6.3±0.2 | 7.2±0.4 * | 6.1±0.3 ** | 6.0±0.3 | 6.3±0.4 | 5.8±0.4 |
Absolute lymphocyte (103cell/µL) | 2.2±0.1 | 2.4±0.1 | 2.1±0.1 | 2.0±0.1 | 2.2±0.1 | 2.0±0.1 |
Absolute neutrophil (103cell/µL) | 3.5±0.2 | 4.1±0.3 * | 3.4±0.3 ** | 3.3±0.3 | 3.8±0.3 * | 3.3±0.3 ** |
Absolute monocyte, basophil, eosinophil (103cell/µL) | 0.61±0.03 | 0.71±0.06 | 0.53±0.03 ** | 0.53±0.03 | 0.55±0.03 | 0.46±0.05 |
CD4+ T-cells (cell/µL) | 815±47 | 830±43 | 822±44 | 739±35 | 710±33 | 730±45 |
CD8+ T-cells (cell/µL) | 676±48 | 779±55 * | 635±44 ** | 609±41 | 671±55 | 630±45 |
Note: The exercise involved a 30-minute session on a stationary bicycle indoors, maintaining an intensity at 75-85% of the maximum heart rate. The values are means ± SE; n=20 per cohort. The p-values indicate the results of a LSD post-hoc analysis. *, p<0.05 compared with pre-exercise value (within the group). **, p<0.05 compared with the post-exercise value (within the group). $, p<0.05 compared with the same time point (in between groups). 0AA, 0 mg of ascorbic acid; 1,000AA, 1,000 mg of ascorbic acid.
3.4. Flow Cytometric Analysis of CD4+ and CD8+ Lymphocytes
Following the exercise, neither the 0AA cohort nor the 1,000AA cohort showed any significant changes in CD4+ T cells (Table 3). In the 0AA cohort, CD8+ T cells were elevated (p<0.05) immediately after exercise and returned to baseline 24 hours later. However, no significant change in CD8+ T cells was observed in participants consuming AA.
3.5. Neutrophil Phagocytosis Assay
The number of yeasts consumed by neutrophils is shown in Fig. (2). After 24 hours post-exercise, the neutrophil phagocytic index in the 1,000AA group showed a slight decrease (p<0.05), but this difference was not statistically significant compared to the 0AA group (p>0.05) (Fig. 3).
4. DISCUSSION
The main findings in this study were that (1) AA counteracted the detrimental effects of high-intensity cycling, which increased an indicator of oxidative stress in sedentary women. (2) Supplementing with AA reduced the increase in white blood cells and CD8+ T cells after exercise, but neither exercise nor AA changed neutrophil phagocytic activity.
Numerous studies indicate that exercise is linked to positive health outcomes, including improvements in cardiorespiratory fitness, muscular strength, bone health, and cardiometabolic health [20-22]. High-intensity exercise has gained popularity, yet an eight-week high-intensity interval training program in young obese females showed no changes in thrombosis markers or fibrin induction [23]. Its effects on exercise-induced oxidative stress, antioxidant status, and immune responses in sedentary adults remain uncertain. Signs of exercise induced inflammation include elevated levels of IL-6, neutrophils, and white blood cells [24]. Inflammatory cells play a dual role in promoting both tissue injury and tissue regeneration. This process is mediated by the coordinated actions of reactive oxygen species, low molecular weight enzymatic antioxidants, growth factors, hormones, and cytokines. These factors work together to maintain a delicate balance between pro- and antioxidants as well as pro- and anti-inflammatory activities [15]. In this study, we found that plasma TAS and SOD levels were not significantly altered by exercise or AA supplementation. According to previous research, increasing the fruit, juice, and vegetable consumption of healthy individuals increases their blood levels of vitamin C but has no effect on their antioxidant status [25]. Zychowska et al. found that 1000 mg of vitamin C supplementation is excessive for healthy elderly women with normal plasma vitamin C levels, especially during a 6-week exercise training period when there are no deficiencies in vitamin C status [26]. It appears that while vitamin C has a strong correlation with antioxidant status, other nutrients and lifestyle choices, such as exercise, play a significant role in maintaining an optimal antioxidant balance [27]. Furthermore, in this study, MDA levels increased significantly soon after exercise, and ascorbic acid successfully mitigated exercise-induced lipid peroxidation. This finding is in line with a previous study that suggested ascorbic acid has powerful antioxidant capabilities, suggesting its potential as a therapeutic treatment to reduce the risk of oxidative stress-related injuries caused by physical activity [28]. A change in the antioxidant capacity of plasma is less important than the protection of lipids from damage. This is due to the fact that lipid damage can cause long-term issues such as chronic inflammation and cardiovascular diseases [29]. In contrast, an increase in antioxidant capacity may only provide short-term benefits.
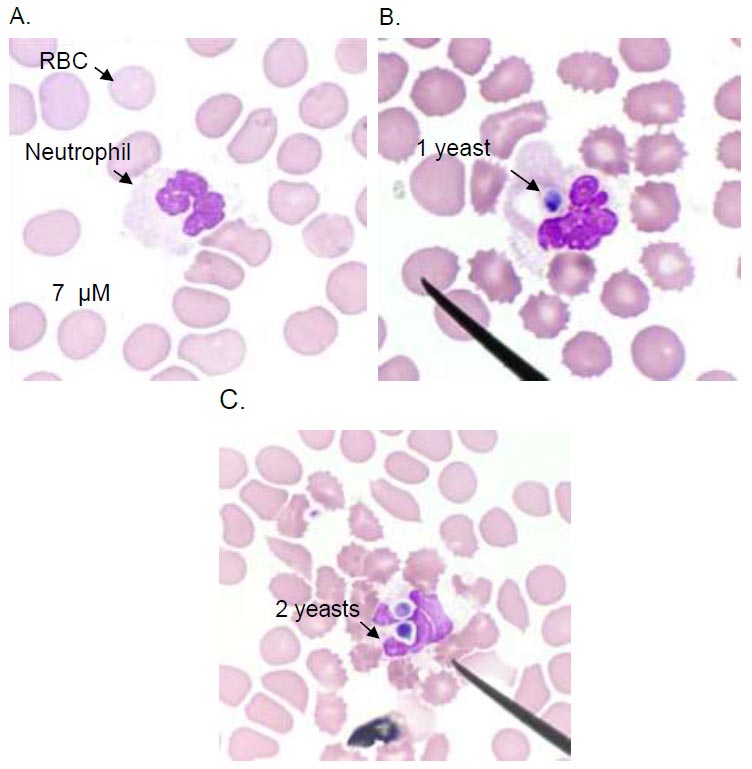
Neutrophil phagocytosis of C.albicans. Light microscopy image of a neutrophil without phagocytosis (A), neutrophils internalize the C.albicans in a phagosome (B and C). Images are representative of sets of different experiments. The scale bar represents 7 µM.
High-intensity exercise has previously been linked to localized inflammation and tissue injury [30]. Our study has demonstrated that high-intensity cycling increases plasma total CK levels, a marker of muscle injury, which are associated with rises in absolute neutrophil numbers and white blood cell counts. These findings are in agreement with a previous study, suggesting that tissue injury may be a contributing factor in the inflammatory response to high-intensity exercise [31]. It has been demonstrated that during acute exercise, peripheral leukocytes are mobilized in a manner that is related to the exercise's intensity and duration [32]. It is believed to be the result of selective redistribution from a marginated pool, which is brought on by an increase in stress hormones after exercise [33]. Cortisol and interleukin-6 work together to promote the migration of neutrophils from the bone marrow into the circulation [31]. The neutrophils that enter the circulation following exercise can increase inflammation, potentially leading to tissue injury [34]. We showed that seven days of ascorbic acid supplementation before exercise had no effect on preventing muscle damage or the rising number of neutrophils in the blood circulation. Krause et al. [35] found no differences between the vitamin C supplementation group (2 g for one week) and the placebo group concerning the phagocytosis and bactericidal activity of neutrophils isolated after high-intensity exercise. It was reported that vitamin C supplementation (1 g/day for two weeks) had no effect on neutrophil oxidative burst or degranulation post-exercise and was equivalent to the placebo group [36]. Hunter et al. found that administering vitamin C supplements (gold kiwifruit provides around 360 mg of vitamin C daily for four weeks) to healthy older adults had no effect on neutrophils' phagocytosis activity [37]. Collectively, these findings suggest that short-term vitamin C supplementation does not improve the function of neutrophils. Our study suggests that the lack of benefit from ascorbic acid supplementation on neutrophil function may be due to the complexity of neutrophil processes, such as chemotaxis, microbe recognition and phagocytosis, and the use of cytotoxic mechanisms to kill pathogens. These mechanisms involve producing reactive oxygen species, releasing antimicrobial peptides, and forming neutrophil extracellular traps. Additionally, the assessment method used for ex vivo neutrophil functions could have influenced the findings.
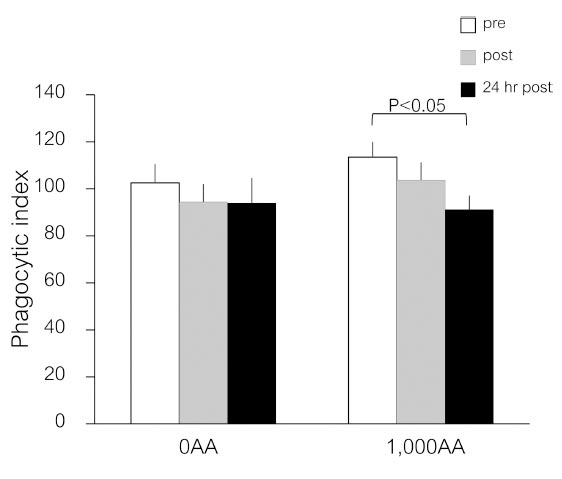
Effects of an ascorbic acid supplement on neutrophil phagocytic function after a single bout of exercise. Values are means ± SE; n=20 per cohort. The p-values indicate the results of an LSD post-hoc analysis.
As a result of exercise, IL-6 is produced in the area of damage. It is then transported to the liver via blood circulation [38]. IL-6 can contribute to persistent inflammation by directing monocytes to the inflamed area [39]. Our study found that high-intensity cycling increases plasma IL-6 levels, but when vitamin C is supplemented, its impact is reduced. Although there were transient changes in monocyte, basophil, and eosinophil counts after exercise, there was no significant increase in the recruitment of monocytes into the bloodstream. Some studies have shown that vitamin C inhibits the generation of IL-6 [16, 40, 41], and prevention of the LPS-induced increase in the number of monocytes generating IL-6 is thought to be the mechanism by which it lowers IL-6 levels [42]. This study provides further evidence of the efficacy of vitamin C in inhibiting the production of IL-6, suggesting that vitamin C supplementation may be beneficial for reducing inflammation associated with high-intensity cycling.
Following a 7-day vitamin C supplement, we observed a significant rise in plasma ascorbic acid levels, indicating that the supplement was effective in increasing vitamin C concentration in the body. Within 24 hours after exercise, there is a temporary rise in the amount of ascorbic acid in the blood. This indicates that vitamin C may have a role in aiding recovery from exercise. According to Gleeson et al., nine men who finished a 21-km race exhibited higher plasma and lymphocyte ascorbic acid levels, and it was hypothesized that ascorbic acid, along with cortisol, may be released into the circulation by exercise from the adrenal glands [43, 44].
We demonstrated that the number of white blood cells and CD8+ T cells rose immediately after exercise and subsequently returned to baseline 24 hours later while taking ascorbic acid seems to lessen this effect. This suggests that taking ascorbic acid before exercise may help to reduce the body's inflammatory response, potentially leading to improved recovery times. Further research is needed to confirm this hypothesis and explore the full implications of these findings. A prior study demonstrated that activated T cells internalize more vitamin C, a phenomenon attributed to increased expression of glucose transporters (GLUT-1 and GLUT-3) [45]. They found that high concentrations of vitamin C (0.25–0.5 mM) during in vitro T cell activation decreased cell viability, decreased the uptake of thymidine, and decreased the release of cytokines. Therefore, the ingestion of 1,000 mg of AA may have helped to limit the number of activated T cells released into circulation in response to exercise. One question is whether taking 1 g of vitamin C every day may weaken any of the adaptations to exercise. According to a prior study, recreationally active males who consumed 1 g of vitamin C orally daily for four weeks while engaging in high-intensity interval training did not experience any reductions in their ability to do physical activity [46]. One potential explanation for how ascorbic acid modulates the immune response induced by exercise is partly attributed to its antioxidant capacity.
In addition, there were no significant changes in plasma glucose, non-esterified fatty acids, or hydroxybutyrate during or after exercise, ensuring that ATP resynthesis is closely matched to the ATP requirement of exercise. However, in the vitamin C supplementation group, plasma glucose slightly increased 24 hours after exercise. In our previous study [13], participants who received ascorbic acid showed a slight increase in plasma glucose 30 minutes after exercise compared to pre-exercise values. These findings indicate that supplementation with vitamin C could contribute to the maintenance of energy metabolism during exercise and recovery, in addition to its role as an antioxidant.
This study has some limitations in that we could not control for every possible lifestyle factor, such as stress levels, diet, and physical activity, that could have had an influence on the study's results, and the individuals with lower or higher fitness levels leave the possibility of residual confounding factors. Additionally, the study only assessed the effects of a seven-day vitamin C supplementation period. Therefore, future research should explore various dosages and durations of vitamin C supplementation before, during, or after exercise. Nevertheless, the results are promising and provide support for further research on the benefits of vitamin C supplementation.
CONCLUSION
In summary, the present study reports that high-intensity exercise increases oxidative stress, muscular damage, and inflammation, along with a temporary increase in CD8+ T cells. Ingesting ascorbic acid before exercise for a week appears to mitigate the exercise-induced increase in MDA level and reduce the migration of white blood cells into the blood circulation while reducing exercise-induced inflammation. Future studies should explore the ideal dosage and duration of vitamin C supplementation, as this will greatly enhance the study's rigor, relevance, and contribution to exercise science and nutritional supplementation.
AUTHORS’ CONTRIBUTIONS
PB conceived and designed the study. PB, VS, and AP carried out all the experimental work and statistical analysis. PB participated in the manuscript design, interpretation, and preparation. All authors read and approved the final manuscript.
LIST OF ABBREVIATIONS
AA | = Ascorbic Acid |
CD | = Cluster of Differentiation |
VO2max | = Maximal Oxygen Uptake |
ROS | = Reactive Oxygen Species |
RNA | = Reactive Nitrogen Species |
SOD | = Superoxide Dismutase |
BMI | = Body Mass Index |
mg | = Milligram |
h | = Hour |
EDTA | = Ethylenediaminetetraacetic Acid |
°C | = Degree Celsius |
g | = Gravity |
ALT | = Alanine Aminotransferase |
IFCC | = International Federation of Clinical Chemistry |
CK | = Creatine Kinase |
MDA | = Malondialdehyde |
TBA | = Thiobarbituric Acid |
TBARS | = Thiobarbituric Acid Reactive Substances |
nm | = Nanometer |
μmol | = Micromole |
CBC | = Complete Blood Count |
DMST | = Department of Medical Sciences Thailand |
C. albicans | = Candida albicans |
NSS | = Normal Saline Solution |
CFU/mL | = Colony Forming Unit Per Milliliter |
FITC | = fluorescein Isothiocyanate |
RD1 | = Phycoerythrin |
PC5 | = Phycoerythrin Cyanine 5 |
µL | = Microliter |
PBS | = Phosphate-buffered Saline |
SE | = Standard Error |
WBC | = White Blood Cells |
IL | = Interleukin |
ETHICS APPROVAL AND CONSENT TO PARTICIPATE
The study and the protocol were approved by the Ethics Research Committee from the Faculty of Associated Medical Sciences, Chiang Mai University (AMSEC-62EX- 040).
HUMAN AND ANIMAL RIGHTS
No animals were used in this research. All procedures performed in studies involving human participants were in accordance with the ethical standards of institutional and/or research committees and with the 1975 Declaration of Helsinki, as revised in 2013.
AVAILABILITY OF DATA AND MATERIALS
All data generated or analyzed during this study is included in this published article.