All published articles of this journal are available on ScienceDirect.
Effect of Additional Buoyancy Swimsuits on Performance of Competitive Swimmers
Abstract
Background:
When in water, the Centers of Buoyancy (CoB) and Mass (CoM) of the human body are positioned cranially and caudally, respectively. With increasing distance between these centers, the sinking torque of the lower limbs increases, with a subsequent decrease in swimming performance due to increased drag.
Objective:
To clarify the effect of additional buoyancy swimsuits on swimming performance.
Methods:
The subjects were eight competitive male swimmers of mean ±SD age 21±2 years. Swimming performance was compared between Conventional (CS) and Additional Buoyancy Swimsuits (ABS). CoM and CoB were identified on land and in water, respectively, with the swimmers maintaining a horizontal posture. CoM was measured by the reaction board method. CoB was calculated as the force exerted in the vertical direction accompanied by changes in inspiratory volume. Swimming velocity and Blood Lactate (BL) concentration value during 200 m front crawl in trials at four different speeds (curve test) were recorded as swimming performance.
Results:
No significant difference in inspiratory volume was observed between CS and ABS (small effect size, d=0.28). The distance between CoM and CoB was significantly shorter for CS than ABS (p < 0.001; large effect size, d=1.08). Both swimming velocity at BL of 4 mmol·L-1 and maximal effort were significantly faster for ABS (p < 0.042; 0.008), with large effect size (d=0.91; 0.98). However, there was no significant difference in maximal BL between CS and ABS (small effect size, d=0.37).
Conclusion:
ABS improves swimming performance by streamlining the horizontal posture.
1. INTRODUCTION
When an object moves through the water, there is an exponential relationship between velocity and drag. When a human is swimming, drag is proportional to the square or cube [1] of the swimming speed. In other words, drag is an important factor influencing improvement in a swimmer’s performance [2-4].
The close relationship between swimming performance and drag can also be understood in terms of differences between Conventional Swimsuits (CSs) used for daily training and those for competitive use. Generally, competitive swimmers use different swimsuits for daily training and for competitive races [5]. Manufacturers also distinguish between swimsuit types, providing highly durable wear suitable for daily use as well as swimsuits that are less durable but enable the athlete to attain the highest levels of performance while supporting their posture and reducing drag. Many studies have evaluated how the major differences between these two swimsuit types affect performance in terms of their materials and shape [6-10].
Three types of drag are generated by the body when swimming: wave-making drag, frictional drag, and pressure drag [7, 8, 11, 12]. Regarding the relationship between swimsuit design and drag, it has been suggested that wave-making drag can be reduced by increasing stroke length during racing [9], although this has not been proven. Frictional drag represents only 10%-15% of total drag [8, 13]. In contrast, pressure drag is largely determined by the frontal surface area when in a horizontal posture [12, 14, 15] and can be expected to improve when a swimsuit is worn [7, 9]. When comparing race swimsuits with CS, the increased coverage of the thighs with competitive swimsuits reduces friction due to skin and muscle, which reduces the frontal surface area [4, 16].
Pressure drag during forward propulsion is influenced by the horizontal posture of the swimmer [4] as well as compression of the body by the swimsuit [7]. When swimmers maintain their horizontal posture in the water, buoyancy acts on them through their center of buoyancy (CoB), and gravitational force acts on them through their center of mass (CoM). Ordinarily, because the CoM is located much more caudally than the CoB, the lower limbs will sink as a swimmer maintains a horizontal posture in the water [17, 18]. In other words, the distance between CoB and CoM (BM distance) is linked to leg-sinking torque: if this distance is shorter, the lower limbs will be closer to horizontal, thus reducing the frontal surface area. Drag will be reduced accordingly, and swimming performance will improve. The banned last-generation swimsuits covered the body from the shoulder to ankle and enabled the establishment of swimming records that exceeded all predictions [19] by allowing the lower limbs to float [20]. Therefore, we could expect that wearing an additional buoyancy swimsuit (ABS) would be useful in supramaximal speed trials of tethered swimming [21] as well as changing the body position of the swimmer in daily training that artificially increases and decreases drag [20]. However, the efficacy of jammer-type swimsuits that add artificial buoyancy has not yet been verified. In addition, CoB and CoM have not been measured for ABSs. Examining the effect of swimsuits with artificially manipulated buoyancy may lead to the development of new training methods by swimmers and coaches, in a similar manner to those developed following research into the effect of changing body position on drag [20].
The present study aimed to clarify the effect of a jammer-type ABS that covers the thighs and has a higher level of buoyancy than that allowed by the Fédération International de Natation (FINA) on swimming performance. We hypothesized that ABSs could improve the posture of swimmers by artificially increasing their buoyancy and lead to higher swimming velocities than are possible with CSs.
2. MATERIALS AND METHODS
2.1. Participants
The participants enrolled in the study were eight competitive male swimmers (age, 21±2 years; height, 1.77±0.07 m; weight, 72.4±8.2 kg). To avoid the effect of sex differences on body mass and distribution of fat influencing the results, all participants were male, and all were collegiate performance swimmers trained in highly repeatable swimming movements. The mean International Swimming Federation (FINA) point score [22] was 539±82. Participation was voluntary, and all swimmers received a detailed explanation of the experiments and the possible risks prior to enrolment in the study. The research procedure was based on the principles of the Declaration of Helsinki, and approval was received from the Institutional Ethics Review Committee of Sendai University before commencing the experiment.
2.2. Swimsuits
Two swimsuit types were used in the current study: CS type for daily training use (box-type, made of polyester and covering the hip joint) and ABS type (Zero Position Professional; Yamamoto Corporation, Osaka, Japan), a rubber jammer style covering the thighs, of thickness 1 mm and buoyancy ~2 N (Fig. 1). Each participant selected their own size, and a supervisor took measurements to ensure that they fit their body perfectly.
2.3. Experimental Design
CoB and CoM in the streamlined posture, blood lactate levels, and swimming velocities were measured for each participant while wearing each type of swimsuit during a set of front crawl incremental step tests (curve tests). The CoB and CoM measurements and curve tests were conducted as a set for each swimsuit type on two consecutive days, with the order of the swimsuit type worn randomized. The interval between the two sets was approximately 24 hours.
2.3.1. CoM and CoB Measurements
CoM was measured using a previously developed apparatus [18, 23] (Fig. 2) with reference to the reaction board method [24]. The system consists of two force plates (LCTA-A-1KN; Kyowa Electronic Instruments, Chofu, Japan) spaced 1.5 m apart on horizontal ground with a wooden frame installed above them. Participants raised their upper limbs to the maximal inspiratory level and paused for 3 seconds while lying supine on top of the wooden frame, with load data recorded on a personal computer with a sampling rate of 100 Hz and analyzed using the CoM Trembling Measuring System (G-Gravity; 4Assist, Tokyo, Japan). Bodyweight (W) was calculated as the sum of the downward vertical force of the centers of the fists and the lateral malleoli on the force plates (hands, F1CoM; feet F2CoM), as shown in the formula below. CoM was then obtained using the following equations:


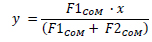
Where x is the distance between the lateral malleolus and center of the fist, y is the distance between the CoM and the lateral malleolus, W is body mass, and F1CoM is the force on the center of the fist. Buoyancy and CoB were measured using the WAKACO apparatus developed by Watanabe et al. [18, 23] (Fig. 3) with reference to McLean & Hinrichs [17]. The WAKACO system consists of a frame placed above the surface of the water in which tension/compression load cells (LUR-A-200NSA1; Kyowa Electronic Instruments) are installed. The cells are positioned directly above the swimmer’s feet and the center of their fists. Wearing a snorkel and nose clip, the swimmer was asked to submerge his entire body underwater and maintain a streamlined posture while holding onto a handgrip, with foot tethers fixed to both ankles, set and maintained at an angle of 90 degrees. The height of the line connecting the hands and feet was then adjusted to be level with the surface of the water as the participant maintained the streamlined position. The depths of the handgrip and foot tethers were set at 20 cm from the surface of the water. To ensure that the swimmer’s body did not break the surface of the water when breaths were taken, weights of 4.5 kg were attached to the handgrip and 2 kg to the foot [18, 23]. All participants were instructed to breathe through the snorkel and keep the nose clip in place to prevent air leakage from any part of the body other than the mouth. Six cycles of controlled breathing were then performed, with the participant engaging in maximum inhalation and maximum exhalation at 5-second intervals. The tip of the snorkel was attached to a pneumotachographic sensor (FM-200XB; Arco System, Kashiwa, Japan) and connected to a dedicated amplifier (FM-200XB; Arco System) to identify pressure differences. The vertical force points (fists, F1CoB; feet, F2CoB) were then obtained at 100 Hz and recorded on a personal computer. The difference in air pressure during inhalation and exhalation (ventilation flow data) was converted into velocity data and output by the amplifier, so we converted these data digitally and recorded them at 100 Hz using the computer. We calculated ventilatory volume by integrating the velocity data. All of the ventilation flow data and load cell data were synchronized to the computer. While participants remained stationary in the water and assuming that all forces acting on the body were balanced, buoyancy B and CoB of the foot area (z) were measured under the same principles as for measurement of CoM, as follows:




CoB and CoM of the participant in the water in the streamlined posture were obtained as follows:

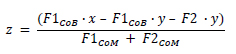
The distance between the CoB and the CoM (BM distance) was obtained using the following formula:

In the analysis of BM distance, the change in buoyancy due to inspiratory volume was normalized, and the value when buoyancy becomes 0 was used (neutral buoyancy), as described previously [18, 23].
2.3.2. Incremental Swimming Step Tests
The incremental swimming step tests (curve test) for determining the swimming velocity at the onset of blood lactate accumulation (OBLA) comprised a set of four 200 m front crawl trials. Similar to previous studies [25, 26], in trials 1–3, participants were instructed to swim at approximately 80%, 85%, or 90% of the speed that resulted in the average swimming times of their best records at 200 m (calculated by dividing the swimming record by the swimming distance); and at maximal effort in trial 4. In trial 1 (80%) to trial 3 (90%), swimmers checked the lap time on the pace clock at each first stroke after breaking out from the flip turn. In trial 4 (maximal effort), the swimmers judged their own maximal effort without adhering to an allocated pace. Blood lactate (BL) concentration values collected from participants’ fingertips were measured at 1-minute intervals after trials 1-3 and at every 2 minutes after the first minute in trial 4 until the maximum value was observed, using specialized measuring equipment (Lactate Pro2, LT-1730; Arkray, Kyoto, Japan). Therefore, the target of analysis in trial 4 was maximum BL (BL@max). Using the method described in previous studies [26, 27], the swimming velocity at BL of 4 mmol·L-1 (V@OBLA) was calculated by plotting the relationship between swimming velocity and BL. The maximal swimming velocity (V@max) was calculated by dividing the swimming record at the maximal effort by the swimming distance. Five minutes was provided as a resting period between each of the four trials.
2.4. Statistical Analysis
Shapiro-Wilk test was used to check for normal distribution of the obtained data. All values were expressed as mean ± Standard Deviation (SD) or as individual values. Paired Student’s t-test was used to compare differences in the same participant when wearing different swimsuit types. The mean difference and respective 95% confidence interval values (95% CI) were also calculated. The effect size was calculated using Cohen’s d, and magnitudes of 0.2, 0.5, and >0.8 corresponded to small, moderate, and large, respectively [28]. The level of statistical significance was set at 5% (p < 0.05).
3. RESULTS
Table 1 shows the comparison of mean inspiratory lung volume at neutral buoyancy, CoB, CoM, and BM distance values between the swimsuits. No significant difference was found between CS and ABS regarding inspiratory volume at neutral buoyancy and CoM, with a small effect size. CoB was significantly higher for CS than for ABS (0.62 cm, p < 0.034), and the effect size was small. BM distance was significantly shorter for ABS compared with CS (0.20 cm, p < 0.001), and the effect size was large.
Table 2 shows the comparison of mean swimming velocity, swimming velocity at OBLA, and BL between the swimsuits. Regarding swimming velocities during the curve test, mean V@OBLA and V@max swimming velocities were both 0.05 m·s-1 faster for ABS (p < 0.042; 0.008), and the effect size of both was large. No significant difference was found between the swimsuits in terms of maximum blood lactate concentration following the curve test, with medium effect size.
4. DISCUSSION
The current study aimed to clarify the effect on swimming performance of a jammer-type ABS with a level of buoyancy higher than that allowed by FINA. The results confirmed that swimsuits with an additional buoyancy of 2 N decrease the distance between CoB and CoM and improve swimming performance.
As air has lower density than water, the greater the amount of breath taken into the lungs when breathing (inspiratory volume), the greater the buoyancy. A previous study that used a similar method to measure the vertical force on swimmers in the streamline posture found that vertical force changed according to inspiratory volume [18, 23]. Therefore, by adding approximately 2 N of buoyancy to swimmers by wearing ABS, we would expect a decrease of air of 0.20 L corresponding to 2 N of buoyancy when the same posture is maintained. However, in the present study, no significant difference in inspiratory volume was observed, for the likely reason that the change in inspiratory volume that accompanies a change in swimsuit differs according to the swimmer. The present findings suggest that the stationary horizontal posture of a swimmer in water is controlled by other factors in addition to inspiratory volume.
Participant | LVN (L) | CoB (cm) | CoM (cm) | BM Distance (cm) | ||||
CS | ABS | CS | ABS | CS | ABS | CS | ABS | |
A | 2.71 | 2.65 | 101.84 | 101.51 | 100.19 | 99.96 | 1.65 | 1.55 |
B | 2.29 | 2.03 | 105.18 | 104.61 | 103.71 | 103.31 | 1.47 | 1.30 |
C | 2.85 | 2.10 | 107.18 | 105.08 | 105.63 | 103.73 | 1.55 | 1.35 |
D | 2.07 | 2.64 | 106.73 | 105.95 | 104.92 | 104.38 | 1.81 | 1.57 |
E | 2.14 | 2.12 | 101.97 | 101.55 | 100.46 | 100.39 | 1.51 | 1.16 |
F | 2.34 | 2.11 | 106.94 | 106.21 | 105.15 | 104.53 | 1.79 | 1.68 |
G | 2.31 | 1.95 | 111.95 | 112.14 | 110.62 | 110.99 | 1.33 | 1.15 |
H | 1.07 | 1.14 | 101.34 | 101.09 | 99.98 | 99.98 | 1.36 | 1.11 |
M ean | 2.22 | 2.09 | 105.39 | 104.77 | 103.83 | 103.41 | 1.56 | 1.36 |
SD | 0.54 | 0.47 | 3.61 | 3.63 | 3.62 | 3.64 | 0.17 | 0.20 |
t Value | 0.967 | 2.631 | 1.772 | 6.978 | ||||
p Value | 0.366 | 0.034 | 0.12 | < 0.001 | ||||
95%CI [LL, UL] | [-0.19, 0.45] | [0.06, 1.18] | [-0.14, 0.99] | [0.13, 0.27] | ||||
Cohen's d | 0.28 | 0.17 | 0.12 | 1.08 |
Participant | V@OBLA (m·s-1) | V@max (m·s-1) | BL@max (mmol·L-1) | |||
CS | ABS | CS | ABS | CS | ABS | |
A | 1.37 | 1.41 | 1.51 | 1.64 | 14.1 | 19.8 |
B | 1.32 | 1.29 | 1.65 | 1.67 | 17.8 | 20.3 |
C | 1.33 | 1.42 | 1.60 | 1.65 | 19.2 | 17.2 |
D | 1.50 | 1.50 | 1.69 | 1.70 | 11.5 | 14.4 |
E | 1.37 | 1.51 | 1.60 | 1.64 | 19.3 | 16.6 |
F | 1.39 | 1.47 | 1.54 | 1.63 | 10.4 | 13.5 |
G | 1.39 | 1.43 | 1.55 | 1.57 | 12.6 | 9.5 |
H | 1.40 | 1.42 | 1.56 | 1.63 | 14.5 | 17.7 |
M ean | 1.38 | 1.43 | 1.59 | 1.64 | 14.9 | 16.1 |
SD | 0.06 | 0.07 | 0.06 | 0.04 | 3.5 | 3.6 |
t Value | -2.480 | -3.705 | -1.028 | |||
p Value | 0.042 | 0.008 | 0.338 | |||
95%CI [LL, UL] | [-0.09, 0.00] | [-0.09, -0.02] | [-4.0, 1.6] | |||
Cohen's d | 0.91 | 0.98 | 0.37 |
The buoyancy exerted on a body when submerged in water depends on the volume of the body. For example, abdominal breathing that lowers the diaphragm and expands the lower chest creates a larger abdominal volume compared with chest breathing, which expands only the upper chest. Accordingly, chest breathing shifts the CoB caudally to a greater degree than abdominal breathing [29]. In contrast, the CoM lies along the length of the body’s longitudinal axis, meaning that there is almost no difference in CoM according to the type of breath; in addition, because BM distance is shorter for abdominal breathing, leg-sinking torque is reduced. A similar phenomenon can be seen for different swimsuits. A previous study of four types of jammer-type swimsuits speculated that the CoB of the body was affected by a miniscule reduction in overall body volume for swimsuits that compressed the thighs more tightly [16]. In the current study, BM distance was significantly shorter (0.20 cm) for ABS compared with CS. This finding indicates that wearing the ABS used in this study assists in maintaining a horizontal posture and makes it more difficult for the lower limbs to sink.
The posture of the swimmer affects swimming performance [2, 12, 14, 15]. When swimming velocity increases, the projected frontal surface area and angle of trunk incline decrease [30]. A previous study reported that regardless of sex or swimming skill, the magnitude of leg-sinking torque is the primary factor in energy expenditure while swimming at maximum lower-limb exercise intensity [14]. A similar study [31] that measured energy expenditure found that leg-sinking torque and drag increased when wearing objects of different densities on the waist. In addition, wearing a triathlon wetsuit raises the position of the swimmer’s body in the water and reduces the frontal surface area, with a consequent improvement in energy costs because of the reduction in drag [32-34]. In this context, the ABS used in the current study reduced BM distance and also significantly improved OBLA swimming velocity. These effects made the swimmer’s posture more horizontal when compared with CS, decreasing the projecting frontal surface area and active drag, which could lead to decreased energy expenditure at a particular constant maximum velocity.
The effect of swimsuit characteristics on swimming performance is influenced by individual differences in a swimmer’s morphology and their swimming skill [35]. In contrast, an increase in artificial buoyancy may cause swimming performance to decrease [36, 37]. Indeed, as observed in the graph plotting the velocities of the present swimmers during the curve tests, swimmers A and D showed a decrease in performance when wearing ABS. However, BM distance was significantly reduced by 0.20 cm. A previous study showed that swimming performance decreased with increased buoyancy after a neoprene band was attached to the lower limbs [38]. This finding suggests that there are likely cases when the advantage that occurs in stationary posture would not, for whatever reason, be linked to an improvement in swimming performance.
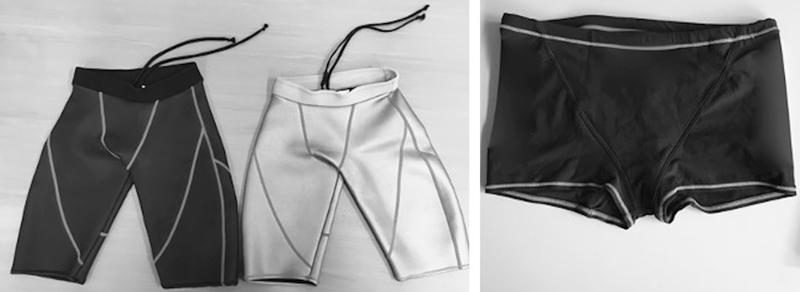
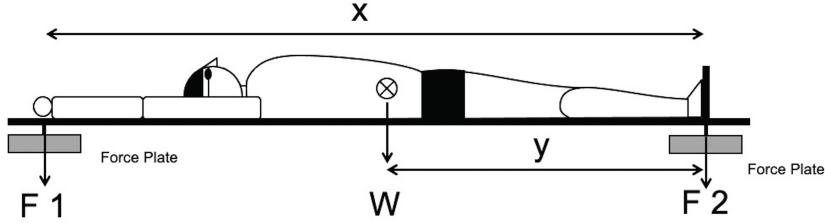
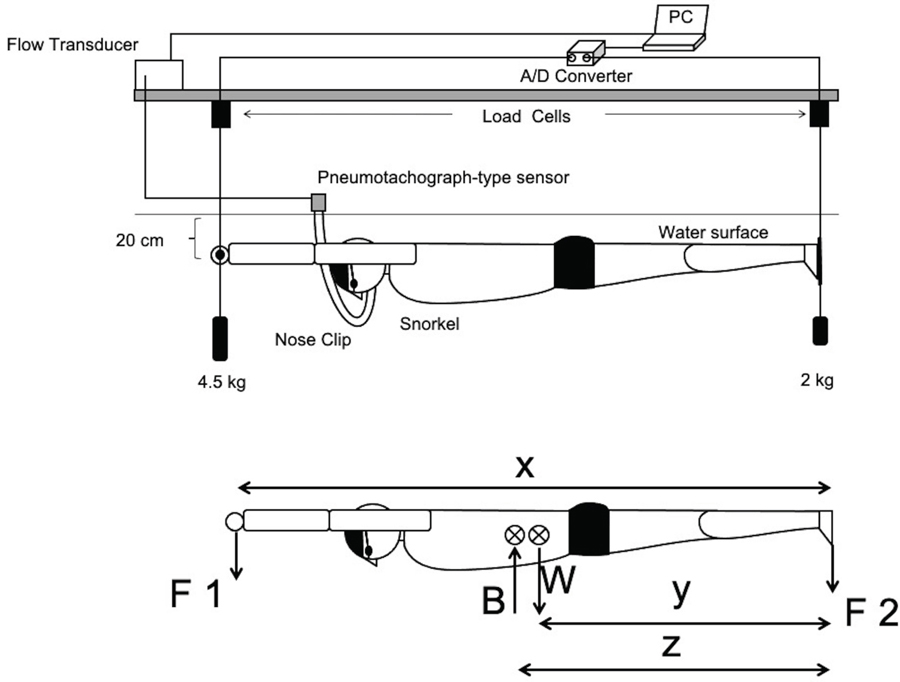
Regarding the limitations of the current study, we did not measure stroke index or drag forces. It is known that wearing a racing swimsuit extends the non-propulsive phase of the stroke and increases stroke length [35]. In addition, the banned last-generation swimsuits reduced the angle of trunk incline and the drag coefficient [20]. To consider the findings of the present study in greater detail, further research of these aspects is required. Moreover, the present sample size was too small to enable a large effect size for statistical power of 0.80 and alpha of 0.05. It is necessary to repeat the study with a greater number of participants to strengthen the validity of the present results.
4.1. Practical Implications
Wearing an ABS resulted in improved swimming performance with reduced effort compared to wearing a CS. The ABS promotes a lower drag posture, which enables the swimmer to move at higher speeds, even at low intensities. This finding indicates that it is possible to check movements (e.g., in practice drills) in body position conditions that are close to competitive race situations. Furthermore, if continuous training while wearing an ABS and maintaining a horizontal posture in the water is linked to improvements in swimming posture, ABS could become a useful swimsuit for daily training use. However, because an overreliance on the posture-improving features of the ABS may be linked to a decrease in the consciousness of how to maintain posture on the part of the swimmer, there is an undeniable risk that this strategy could, in fact, damage performance. Accordingly, when using ABS, both coaches and swimmers should focus on the individual effects of the ABS on performance.
CONCLUSION
Our research found that adding buoyancy of 2 N to a swimsuit enabled improvements in swimming performance by decreasing the distance between CoB and CoM and by promoting a posture with reduced lower-limb torque. It is important to note that the effects of the ABS may vary according to the individual swimmer.
ETHICS APPROVAL AND CONSENT TO PARTICIPATE
This study was approved by the Institutional Ethics Committee of Sendai University, Japan with approval number 29-18.
HUMAN AND ANIMAL RIGHTS
No animals were used in this research. All human research procedures were followed in accordance with the ethical standards of the committee responsible for human experimentation (institutional and national), and with the Helsinki Declaration of 1975, as revised in 2013.
CONSENT FOR PUBLICATION
All participants signed informed consent prior to participating in the study.
AVAILABILITY OF DATA AND MATERIALS
Not applicable.
FUNDING
This work is supported by the JSPS KAKENHI, grant number 16K01713.
CONFLICT OF INTEREST
The authors declare no conflict of interest, financial or otherwise.
ACKNOWLEDGEMENTS
Declared none.