All published articles of this journal are available on ScienceDirect.
Effects of Static and Dynamic Stretching on Force Sense, Dynamic Flexibility and Reaction Time of Children
Abstract
Background:
Traditionally, stretching protocols are basic components of warm-up aiming to improve performance and reduce injuries. However, the literature suggests that different stretching protocols during warm-up may have diverse effects on performance.
Objective:
The purpose of this study was to compare the acute effects of three different stretching protocols on force sense, dynamic flexibility, reaction time and movement time.
Methods:
The study included twenty-five participants who were TaeKwonDo young male players (age = 11.78 ± 1.66 years.). All the participants performed one of the following protocols on different days: (a) 5 min jogging followed by 3 min Static Stretching (SS), (b) 5 min jogging followed by 3 min Dynamic Stretching (DS), and (c) 5 min jogging followed by 3 min of rest (NS). After the protocols, the participants performed the following measurements: (a) force-matching test at 20% maximal isometric voluntary contraction (force sense), (b) active straight leg raise test (dynamic flexibility) and (c) reaction and movement time test.
Results:
Repeated measures analysis of variance revealed no significant main effects on force sense. Furthermore, SS performed significantly better in dynamic flexibility in comparison to NS, and DS performed significantly better in terms of dynamic flexibility and movement time compared to SS.
Conclusion:
According to the results of the study, it seems that force sense is not affected by either SS or DS protocols (30 sec duration per muscle group). Moreover, it seems that DS in the warm-up is more appropriate than SS for activities requiring dynamic flexibility and movement time.
1. INTRODUCTION
Traditionally, athletes used to follow pre-activity routines which include various stretching modes (e.g. static stretching exercises), because this activity thought to improve athletes’ performance and reduce the chance of injuries [1]. However, recent literature suggests that different stretching modes during warm-up may have diverse effects on physical performance [2]. For example, previous studies reported that Static Stretching (SS) may increase flexibility [3], but at the same time, it may also decrease force output [4]. On the other hand, although several studies reported that Dynamic Stretching (DS) has no detrimental or positive effects on force output [5], recent studies reported that DS induced impairments on force [6] and flexibility [7]. Therefore, the challenge for trainers and researchers is to seek for the appropriate warm-up stretching modes.
The stretch-induced decreases in force have been attributed to two mechanisms: (a) changes in the musculotendinous unit (mechanical factors) and (b) impairments in neural output (i.e. decreased motor unit activation and altered reflex sensitivity) [8]. Regarding the mechanical factors, studies reported that SS reduces Musculotendinous Unit (MTU) stiffness which may negatively alter the length-tension relationship, resulting in a lower rate of force production. With regard to the second mechanism, a number of studies demonstrated that SS reduces neural drive, which may lead to an inhibition of optimal muscle activation [9].
Since SS influences neural activation and results in acute changes in force production; it may also affect the force sense. Force sense is considered to be a component of proprioception and is defined as the ability to accurately reproduce a given force during a voluntary contraction [10]. This sense of force enables us to estimate the magnitude of force production appropriate for the performance of a task. Golgi tendon organs are located at the ends of muscle fibers, that are principally contraction receptors (i.e. monitor muscle tension) and are considered to be the major receptors responsible for conveying information regarding force sense [11]. Therefore, changes in Golgi tendon organs sensitivity, due to SS, are expected to lead to alterations in sensory information regarding force sense.
Although the importance of force sense to sports activities is indisputable, studies regarding warm-up effects on proprioception have focused mainly on position and movement sense [12]. The few studies examining the effects of SS on force sense reported no significant differences between control and SS conditions [12, 13]. However, these studies used SS durations that are not practiced in sports activities and did not examine the effects of Dynamic Stretching (DS) on force sense.
In addition, the participants of the studies investigating the effects of SS on force sense were adults [12, 13]. To our knowledge, no study investigated the impact of SS and DS on force sense in children. The structure of children’s musculotendinous unit is more than that of adults [14], consequently, children may show a different reaction to stretching compared to adults. Therefore, the first aim of this study was to compare the effects of static and dynamic stretching modes on force sense of young children.
Besides force sense, Reaction Time (RT) and Movement Time (MT) are also crucial factors for successful participation in sports activities and pre-performance SS may have a negative effect on them. Several researchers suggest that SS increases the flexibility of the muscle-tendon unit and this may impair force production and influence neural activation patterns [15]. As a result, acute changes in neuromuscular activation following stretching may affect RT and MT. Despite their importance in sports activities, only a limited number of studies have investigated the effects of stretching on RT and MT. Specifically, Behm et al. [13] reported impairment in RT and MT after SS, whereas Alpkaya and Koceja [16] and Chatzopoulos et al. [17] reported no significant effects of SS. The contradictory results it necessary to further investigate the issue.
Dynamic flexibility refers to the active range of motion within the full range of motion in a joint [18]. Some studies reported that DS provides similar flexibility compared to SS [19], while other studies have shown less improvement [7]. However, although performance demands mainly a high level of dynamic flexibility, most studies examined the effects of stretching on static flexibility [20]. The few studies examining the effects of stretching on dynamic flexibility reported conflicting results [21, 22]. For instance, Dalrymple et al. [21] found that SS greater improvements in dynamic flexibility of dancers than DS, whereas Amiri-Khorasani et al. [22] reported that professional soccer players performed better after DS. Therefore, further studies are needed to determine whether the dynamic or static stretching during warm-up is more appropriate to enhance dynamic flexibility.
Force sense, RT, MT and dynamic flexibility are crucial components for successful participation in sports activities. Therefore, it is important for athletes to apply pre-activity stretching modes that enhance and not impair their performance. The purpose of the present study was to compare the acute effects of SS and DS on force sense, dynamic flexibility RT and MT of young children. It was hypothesized that SS would increase dynamic flexibility and impair force sense, RT and MT.
2. MATERIALS AND METHODS
2.1. Participants
Twenty-five TaeKwonDo young male players with no history of lower limb injury or disease volunteered to participate in this study (age 11.78 ± 1.66 years, body mass 46.50 ± 9.20 kg, height 153.52 ± 10.07 cm, training years 5.34±2.20, and training unit/week 3.84±.94). The study was conducted in accordance with the ethical guidelines of the local university and all the procedures followed the latest version of the declaration of Helsinki. Informed consent was obtained from the guardians and the participants, and they could withdraw from the study at any time.
2.2. Procedures
Before data collection, the participants attended one orientation session, in which they were familiarized with the stretching procedures and the performance measures. All protocols began with 5 min jogging at a self-selected moderate intensity. After the aerobic activity, the participants performed either 3 min static stretching or 3 min dynamic stretching, whereas in the non-stretching protocol (control), they sat quietly for 3 min. After completing one of the protocols, all the participants performed the test measures. All the study procedures took place between 17:00–19:00 hr.
2.2.1. Static Stretching Protocol
Static stretching exercises included: quadriceps stretch (Fig. 1a), hamstring stretch (Fig. 1b) and iliopsoas stretch (Fig. 1c). Each stretch was held for 30 sec at a point of mild discomfort and the contralateral muscle group was stretched after a period of 10-15 sec so that the participant could change position [23]. The total SS time was 3 min (±1 min).
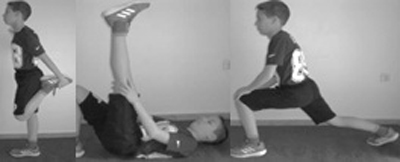
2.2.2. Dynamic Stretching Protocol
The exercises of the DS protocol stretched the same muscle groups as in the SS protocol: Quadriceps, hamstrings and iliopsoas (Fig. 2). Participants performed the dynamic stretches for 30 sec at a rate of approximately 1 stretch cycle every 2 seconds, however, this inevitably varied due to the range of movements performed by each participant [23]. Each exercise was performed 5 times slowly and then 10 times as quickly as possible in a controlled manner without bouncing [23]. Participants were instructed to try and attain the maximal Range of Motion (ROM) during dynamic stretching. The same 15 sec rest period was taken between exercises as in the SS protocol. Total DS time was 3 min (±1 min).
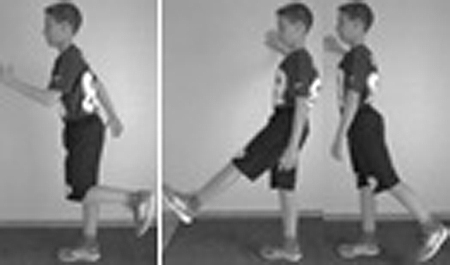
Quadriceps stretch (Fig. 2a): In the standing upright position, the participant contracted the hamstrings and flexed the leg so that the heel touched the buttock.
Hamstring (Fig. 2b): Participants used a wall to stabilize the body during the stretch. In the standing upright position, the participant lifted one extended leg and then returned to starting position.
Iliopsoas (Fig. 2c): Participants used a wall to stabilize the body during the stretch. They flexed the hip and knee as close to the chest as possible and when maximum knee height was attained , they brought forcefully the hip into extension. In order to isolate the iliopsoas, maintenance of the upright trunk posture and avoidance of internal and external rotation of the hip throughout the motion were stressed.
2.3. Measurements
All measurements were conducted with the dominant leg.
2.3.1. Force Sense
The Iliopsoas muscle is the most important muscle in order to lift the leg and attack or defend in TaeKwonDo (e.g. Kicks to the front). In order to measure force sense of iliopsoas, force-matching procedures were followed at 20% maximal isometric voluntary contraction. The iliopsoas’ Maximal Voluntary Contraction (MVC) was measured with an isokinetic dynamometer (Cybex Norm; Cybex,USA) one week prior to the study (Fig. 3).
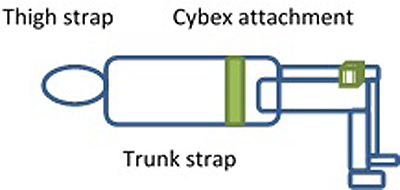
The participants laid down on a bed in the supine position, with the trunk and left thigh fixed by a strap at a hip joint angle of 180° and knee joint flexed at 90° [24]. The MVC test was sustained for 5 s and two trials were performed with 90 s rest. Participants were encouraged verbally to exert the highest possible force during the test. If there was more than a 5% difference in maximum force output, another trial was performed. The trial with the highest MVC value was used for calculation of 20% MVC [12].
The matching force task used the same set-up as the MVC test. Participants were asked to exert sufficient isometric force over a 5-s period to match the 20% of the MVC. Visual feedback was always given for the first two trials, while the computer screen was obstructed from view for the subsequent three trials [13]. The difference between the target force (20% of MVC) and the average of the three trials (without feedback) produced in absolute value was calculated and used for analysis.
2.3.2. Dynamic Flexibility
Dynamic hamstring flexibility was measured with an electro-goniometer (.02° accuracy, sampling frequency 100 Hz, www.vernier.com) using the active straight leg raise test [21]. The greater trochanter was used as a reference point for the axis of the goniometer and the lateral femoral epicondyle was the other reference point (0o). The participant lifted the leg as high as possible while keeping the knee extended two times, and the mean of these measurements was used for data analysis.
2.3.3. Reaction Time and Movement Time
RT and MT were measured using a reaction timer apparatus (Lafayette Instruments Co., model 63017). The apparatus comprises a start and a stop button which are situated on the floor (50 cm distance). The task entailed movement of the dominant foot in response to the illumination of a light bulb. From a standing position, the participants pressing the start button with their dominant foot. Upon illumination of the light bulb, the participants released the start button and moved the foot forward to touch the stop button (50 cm). This action involves hip flexion (iliopsoas) and knee extension (quadriceps). The RT is defined as the time between the light stimulus and the release of the start button. The MT is defined as the time between the initiation of movement (release the start button) and pressing the stop button. Participants performed one practice attempt and three trials with 30 sec rest periods.
Prior to the study, a test-retest of 48 hours between measurement of all the analyzed variables was performed with 14 TaeKwonDo players (age 12.8 ± 2.3 years). The reliability Intraclass Correlation Coefficient (ICC) of force sense was .71, dynamic flexibility .73, reaction time .70 and movement time .78. The participants of the test-retest were not included in the study.
2.4. Statistical Analysis
In order to investigate differences between the three protocols, data were analyzed using one-way Analysis of Variance (ANOVA) repeated measures. Post hoc analyses were conducted using Bonferroni pairwise comparisons. The distribution of all the variables was examined using the Shapiro-Wilk test and no significant difference was found. Cohen’s d effect size was calculated according to the formula
. All statistical analyses were conducted using SPSS (version 20) and significance was set at p ≤ .05.
3. RESULTS
Descriptive statistics of the dependent variables are presented in Table 1.
No stretching | Static | Dynamic | |
---|---|---|---|
Force sense (N) | 7.76 (3.64) | 8.85 (6.26) | 9.61 (5.77) |
Flexibility (degrees) | 112.3 (14.91) | 118.63 (13.29) † | 122.32 (13.21)* |
RT (ms) | 396.78 (63.45) | 403.52 (77.01) | 385.58 (76.13) |
MT (ms) | 751.90 (131.27) | 757.37 (123.32) | 703.21 (113.98) * |
†Significant difference from no stretching protocol (p<.05).
3.1. Force Sense
Regarding force sense, repeated measures ANOVA showed that there were no significant differences between the three stretching modes (F=.763, p=.472, partial eta squared ηp2=.031).
3.2. Dynamic Flexibility
Repeated measures ANOVA indicated a significant difference between the protocols (F=16.204, p<.001, partial eta squared ηp2=.403). Bonferroni correction revealed that the SS was significantly better than the NS (t=2.87, p=.008, 95% CI [1.77, .10.87], Cohen’s d=.44). Moreover, the DS group was observed to be significantly better than the SS group (t=2.81, p=.01, 95% CI [.98, 6.39], Cohen’s d=.27), and the ΝS group (t=5.85, p=.00, 95% CI [6.49, 13.55], Cohen’s d=.71).
3.3. Reaction Time
Repeated measures ANOVA indicated no significant difference between the protocols (F=.755, p=.476, partial eta squared ηp2=.03,
3.4. Movement Time
Repeated measures ANOVA indicated a significant difference between the stretching modes (F=7.16, p=.02, partial eta squared ηp2=.23). Bonferroni correction revealed that the DS group was significantly better compared to the SS group (t=3.29, p=.003, 95% CI [20.26, 88.05], Cohen’s d=.61), and the ΝS group (t=2.91, p=.008, 95% CI [14.25, 83.13], Cohen’s d=.39).
4. DISCUSSION
The purpose of the present study was to compare the effects of static and dynamic stretch protocols on force sense, dynamic flexibility, RT and MT in young children. The results showed that neither static nor dynamic stretching had an effect on force sense and RT. Moreover, both SS and DS showed better results for dynamic flexibility compared to no-stretching, however, DS resulted in significantly greater dynamic flexibility than SS. In addition, DS was observed to be more effective in terms of MT compared to SS.
There is evidence that SS increases the compliance of the muscle-tendon unit [25] and alters neural activation of Golgi tendon organs [4]. Therefore, since SS alters neural activation of Golgi tendon organs, it was expected that it would lead to an increase in the error of force sense. However, according to the findings of the present study, there was no significant difference between the stretching protocols.
The results of the present study are in agreement with those of Behm et al. [13] and Torres et al. [12]. It seems that changes in Golgi tendon organs sensitivity imposed by stretching are not sufficient to affect the force sense. An explanation could be that the inculcation of force sense is not solely based on the information coming from the periphery (Golgi organs). It is believed that force sense is also generated by signals of central origin associated with motor commands [11], which implies that the peripheral input of force sense is always reafferent in origin. Therefore, since force sense is generated in a central sensory area, it is possible that the contribution of motor command signals compensates the altered signals, due to stretching, originating in the periphery and results in the appropriate force sense [4]. Moreover, sensory inputs may not be restricted to Golgi tendon organs and that peripheral receptors from cutaneous and articular tissues also contribute to afferent proprioceptive information [26]. This implies that sensory information conveyed by other than Golgi tendon organs receptors may be sufficient to maintain normal levels of force sense even after stretching. Apparently, more research is needed to clarify this issue.
According to the results of the current study, both SS and DS had a statistically significant effect on dynamic flexibility, but DS resulted in greater dynamic flexibility compared to SS. Therefore, it seems that the mechanisms responsible for acute increases in flexibility after SS and DS are not the same. It is hypothesized that repeated muscle contraction during DS increases muscle temperature and this may decrease the viscous resistance, leading to an enhancement in muscle extensibility [27]. On the other hand, acute increases after SS are attributed to increased tolerance to stretch and/or to changes in mechanical properties of the muscle-tendon unit (i.e., reduced muscle stiffness) [28]. Moreover, the better results of DS compared to SS on dynamic flexibility could be attributed to different levels of force that is produced after the two stretching modes. The performance in active straight leg test is strongly affected by the force of the agonist muscles [29], and several studies reported that DS results in higher force performance than SS [2]. Therefore, it is possible that DS, compared to SS, affected hip flexors to perform a stronger motion of the limb resulting in higher dynamic flexibility.
A limited number of studies could be found that compared the effects of static and dynamic stretch on dynamic flexibility [22, 30]. In the study of Amiri-Khorasani et al. [22], male soccer players showed better dynamic flexibility after DS, whereas Morrin and Redding [30] reported that women dancers performed better after SS. An explanation of the divergent findings could be the different gender of the studies’ sample. Several studies demonstrated a lack of performance decrement in women after SS [21]. Women display lower musculotendinous stiffness compared to males and perhaps are less affected by SS because of their inherent lower stiffness [31]. Apparently, there is a need for further studies to understand the effects of SS and DS on dynamic flexibility.
In relation to MT, the present study found that the DS induced better results than the SS. However, Chatzopoulos et al. [17] reported no significant differences between SS and DS. The different findings may be attributed to the different stretching durations. In the current study, the duration of the stretching modes was 30 sec, whereas in the study by Chatzopoulos et al. [17], it was 15 sec. Ιt is well established that static stretching-induced performance decreases are dependent on stretch durations; the longer the stretch duration, the greater the performance reductions [2]. Concerning dynamic stretching, it seems that shorter durations of dynamic stretching do not adversely affect performance, and longer duration of dynamic stretches (> 30 repetitions) may facilitate muscular performances [23]. Therefore, the better results of the present study may be attributed to the greater duration of DS (30 sec) compared to the 15 sec reported by Chatzopoulos et al. [17]. Considering the few studies which have compared the effects of DS and SS on MT, we should be cautious regarding the potential effects of the different treatments on MT.
CONCLUSION
The results of the present study demonstrated that neither SS nor DS has interference in force sense performance. Consequently, 30 sec SS or DS per muscle group does not constitute sufficient influence on the muscle receptors function, which could compromise force sense. Moreover, SS prior to sports practice improves dynamic flexibility but does not influence RT and MT. On the other hand, DS participants performed significantly better on dynamic flexibility and MT, compared to SS. Therefore, the proposition is to prefer DS than SS in pre-performance activities requiring dynamic flexibility and MT.
ETHICS APPROVAL AND CONSENT TO PARTICIPATE
The study was conducted in accordance with the ethical guidelines of the Aristotle university.
HUMAN AND ANIMAL RIGHTS
No animals were used in this research. All human research procedures followed were in accordance with the ethical standards of the committee responsible for human experimen- tation (institutional and national), and with the Helsinki Declaration of 1975, as revised in 2013.
CONSENT FOR PUBLICATION
Informed consent was obtained from the guardians and the participants, and they could withdraw from the study at any time.
AVAILABILITY OF DATA AND MATERIALS
The data supporting the findings of the article is available in the “figshare” athttps://figshare.com/articles/The_Open_ Sports_sav/9201761, reference number https://doi.org/10.6084/ m9.figshare.9201761.v1.
FUNDING
None.
CONFLICT OF INTEREST
The authors declare no conflict of interest, financial or otherwise.
ACKNOWLEDGEMENTS
Declared none.