All published articles of this journal are available on ScienceDirect.
Biomechanical Analysis of the Knee Joint Load During a Unilateral Sit-to-Stand Movement
Abstract
Background and Objectives:
Sit-to-Stand (STS) movements are fundamental activities of daily living. As STS movements can be physically demanding especially for the elderly, bi- and unilateral STS movements are frequently used in motor tests to measure lower limb strength. In contrast to bilateral STS movements, the knee joint loads occurring during unilateral STS movements as well as the influences of chair height or lower limb dominance are still unknown.
Methods:
In a randomized study approach knee joint loads during unilateral STS movements from three different chair heights have been analyzed using biomechanical motion analysis in a population of 19 healthy middle-aged adults. Additionally, the influence of lower limb dominance and the level of perceived exertion have been investigated.
Results:
Lower limb dominance had no effect on knee joint load. In contrast, chair height significantly affected the peak shear forces in anterior (high: 3.94 ± 0.63 N/kg; low: 4.09 ± 0.61 N/kg) and lateral (high: 1.52 ± 0.79 N/kg; low: 1.78 ± 0.88 N/kg) direction as well as the peak knee adduction moment (high: 0.56 ± 0.29 Nm/kg; low: 0.65 ± 0.32 Nm/kg). Additionally, chair height but not limb dominance significantly affected the level of perceived exertion (high: 11.1 ± 2.8; low: 12.5 ± 3.5).
Conclusion:
The detected knee joint loads occurring during a unilateral STS movements are similar to those of other activities of daily living like e.g. stair ascent and thus, unilateral STS movements are applicable for usage in motor tests for middle-aged subjects. While lower limb dominance has no impact on the knee joint load, lower chair heights increase the load on the knee joint. Therefore, chair height should be considered when using unilateral STS movements in motor tests.
1. INTRODUCTION
An important aspect of sports science is the analysis of human physical performance. Various methods are used for this purpose, including instrumented and motor performance tests, which measure the differences between various personal traits (e.g. motor ability or motor skill). Compared to instrumented test procedures as used in the fields of sports physiology and biomechanics, motor tests are less precise [1], but more feasible and require less specific diag-nostic knowledge. Nonetheless, motor performance tests are scientific methods that must meet the test-theoretical quality criteria and be sufficiently sensitive to be able to test the underlying theoretical model [2].
Motor tests are often used in the field of elite sports for e.g. talent selection [3] and performance diagnostics [4]. They are also increasingly applied in the field of health-related sports as well as sports for the elderly [5]. These tests determine performance in basic abilities such as strength and endurance, which can have a beneficial impact on health if manifested to a certain degree [6, 7]. Motor tests in the field of health-related sports or sports for the elderly are often derived from Activities of Daily Living (ADLs) [8], which aim to detect a person’s capability to meet the practical demands of everyday life. One example of these ADL-derived test tasks is the group of Sit-to-Stand (STS) movements, which have a high relevance in various activities of everyday life [9, 10] since they are performed on average 60 times a day [11].
As well as being an important part of everyday life, the STS movement is a challenging movement for elderly individuals [12] due to decreased strength of the knee extensor muscles [13]. Therefore, the STS movement is frequently incorporated in motor tests with varying degrees of difficulty to determine the strength of the lower limbs. Examples of motor tests including STS movements with bilateral execution are the 5-Repetition STS test [14], the Timed Up & Go test [15] as well as the 30-second chair test [16]. A motor test including unilateral STS movements is the European Fitness Badge [17]. For widespread applicability of the STS movement as a diagnostic tool in the field of health- or age-related sports, the scalability of task difficulty is of particular importance. With advancing age, motor performance develops multidirectional, leading to an increased inter-individual variability in the diverse dimensions of motor performance [18]. This trend can already be observed in middle adulthood (35-65 years) [6] and continues throughout late adulthood (> 65 years) [19].
In the context of a motor test, STS movements generally have three degrees of freedom that can be manipulated to account for the heterogeneous developmental processes, and subsequently to avoid ceiling effects: (I) chair height, (II) unilateral or bilateral execution and (III) number of repetitions in a given time period. However, it was previously shown that bilateral STS movements from decreasing chair heights can cause high loads, especially in the knee and hip joints [20, 21], which probably also applies to unilateral or repeated executions of an STS movement. Additionally, it should be considered that the musculoskeletal system of middle-aged and old adults often show impairments such as osteoarthritis, with the highest prevalence at the knee joint [22, 23]. Against this background, it is crucial to consider the load applied to the lower extremities – especially the knee joint – of middle-aged or elderly subjects while performing STS movements within a motor test.
Knee joint load during bilateral STS movements has been extensively studied in the literature [24-26]. It is well documented that chair height decisively influences the difficulty of the movement task and lower chair heights lead to significantly increased moments at the knee joint, resulting in an increased knee joint load [13, 20, 21, 27]. However, no studies have investigated knee joint load during unilateral STS movement of subjects in middle-to-late adulthood. It was shown by Yamako et al. [28] that 89.5% of men and 71.0% of women aged 60-70 are able to perform a unilateral STS movement. Given the increased inter-individual variability in motor abilities of subjects in mid and late adulthood, the level of difficulty and the resulting joint loads must be considered when using STS movements as a motor test.
Additionally, several studies found an uneven load distribution between the lower limbs during the bilateral STS movement [29-31] as well as asymmetries in the knee extension muscles of the dominant and non-dominant limb [32, 33]. Therefore, lower limb dominance should be considered when conducting biomechanical analyses of unilateral STS movements.
The present study aims to fulfill the research gap by analysing knee joint loads in a unilateral STS movement in a population of healthy, middle-aged adults under consideration of different chair heights and lower limb dominance.
2. MATERIAL & METHODS
2.1. Participants
A total of 19 subjects participated in this study (age: 52.2 ± 5.1 years; height: 173 ± 10 cm; weight: 71.8 ± 12.1 kg; BMI: 24.0 ± 2.9; 12 females). The age group was selected based on studies showing a decrease in strength abilities after the age of 40 [32] and though to maintain the feasibility of the movement task for the subject population [28]. Subjects were excluded from participation if they suffered from any self-reported pain, limited mobility in the lower extremities or any kind of balance disorder. The study was approved by the Institutional Review Board. All participants were informed about the protocol and gave their written informed consent before participating in the study.
2.2. Study Design
The research questions were addressed using a cross-sectional study design with subjects performing unilateral STS movements from three different chair heights as well as their dominant and non-dominant limb. Based on an extensive internet research on commercially available chair heights of different manufacturers, the low chair height was determined at 42 cm and the high chair height at 47 cm. However, if the chair height of 42 cm resulted in a knee flexion angle below 90°, both chair heights were increased by 5 cm resulting in a low chair height of 47 cm and a high chair height of 52 cm. An individual chair height resulting in a knee angle of 90° was added for enhanced inter-subject comparability. The mean chair height in this condition was 44.4 ± 3.5 cm.
2.3. Study Procedure
Following an independent warm-up, subjects were given standardized instructions regarding the execution of the task and were permitted to perform a practice trial. The subjects then performed a unilateral STS task under six different conditions (Fig. 1) in a randomized order. For each condition, three consecutive trials were recorded with a resting period of one minute between each trial and three minutes between each condition, resulting in a total of 18 trials per subject. Following each trial, the subjects were asked to rate their level of perceived exertion on the Borg scale [34] ranging from 6 (no exertion) to 20 (maximal exertion). Unsuccessful trials were classified as maximal exertion.
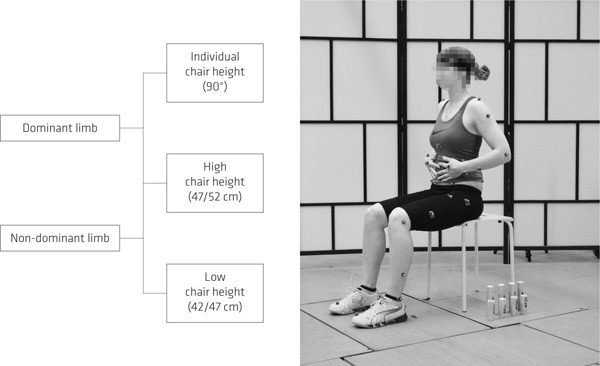
The seating position was standardized by only allowing chair contact with the buttock (crotch at the anterior chair edge). The feet were positioned so that the shank was perpendicular to the ground. Both the seating and foot positions were marked to allow consistent positioning throughout all trials and conditions. The hands rested on the trunk during the entire trial to prevent active use of the upper limbs (Fig. 1). Subjects were equipped with standardized footwear (Puma Vellum).
2.4. Data Acquisition and Processing
3D biomechanical motion analysis was conducted to quantify knee joint loads during the STS movement. A 12-camera motion capture system (Vicon Motion Systems Ltd., Oxford, UK) with a recording frequency of 200 Hz and a force plate (AMTI, Watertown, Massachusetts, USA) with a recording frequency of 1000 Hz were used for data acquisition. Data from all valid trials were processed using Vicon Nexus software (v 1.8.5) and marker trajectories were filtered using a 4th order Butterworth filter with a cut-off frequency of 10 Hz. The Centre of Mass (COM), knee moments and forces were calculated using the Vicon Plug-in Gait model [35]. Peak external knee moments and shear forces were normalized to body weight and served as dependent variables for the quantification of knee joint load.
Trials were considered invalid and excluded from analysis if no stable upright standing position (knee flexion angle < 25°) was achieved prior to foot strike of the contralateral limb, or if the arms were removed from the trunk. After exclusion of invalid trials, one trial of each condition was selected for analysis for each subject (2nd trial for 2 or 3 valid trials, 1st trial for one valid trial).
Movement initialization was determined as the first instance of the COM crossing the anterior chair edge. Movement termination was defined as no further extension of the knee joint. Seat-off was defined when contact with the chair ended and was detected by means of the vertical ground reaction force corresponding to 100% of the subject’s body weight [9]. This detection method demonstrated reasonable accuracy during a validation trial using two force plates: a discrepancy of 0.017 (± 0.011) seconds was found compared to actual seat-off.
2.5. Statistical Analysis
All statistical tests were performed using IBM SPSS Statistics (v 24.0). Data were analysed for normal distribution using a Shapiro-Wilks normality test and for sphericity using Mauchly’s test. If sphericity was violated, a Greenhouse-Geisser correction was used. Four repeated-measures two-way ANOVAs were conducted to assess the effects of chair height and lower limb dominance on each of the dependent variables. In addition, effect sizes (ƞ2p) were calculated for main and interaction effects. Significant results of the ANOVAs were analysed in post hoc analyses with Bonferroni adjustment for multiple comparisons. Data of perceived exertion were analysed for differences due to chair height and lower limb dominance using a Friedman test and a Wilcoxon signed-rank test, respectively. Values of p < 0.05 were considered statistically significant.
3. RESULTS
Of the 19 subjects, 17 were able to successfully conduct the unilateral STS movement under all imposed conditions. Only two subjects were unable to perform the unilateral STS movement in the low and 90° conditions.
An overview of the peak knee shear forces and moments (means ± SD) during the unilateral STS movement under various conditions is given in Table 1.
90° | High | Low | |||||
---|---|---|---|---|---|---|---|
Dominant | Non-dominant | Dominant | Non-dominant | Dominant | Non-dominant | ||
Shear forces (N/kg) |
Anterior | 4.19 ± 0.76 | 4.00 ± 0.58 | 3.98 ± 0.62 | 3.90 ± 0.66 | 4.01 ± 0.67 | 4.17 ± 0.57 |
4.10 ± 0.67 | 3.94 ± 0.63 | 4.09 ± 0.61* | |||||
Lateral | 1.94 ± 1.08 | 1.63 ± 0.78 | 1.61 ± 0.80 | 1.43 ± 0.81 | 1.79 ± 1.02 | 1.77 ± 0.78 | |
1.79 ± 0.93* | 1.52 ± 0.79 | 1.78 ± 0.88* | |||||
Moments (Nm/kg) |
Flexion | 1.49 ± 0.25 | 1.52 ± 0.30 | 1.50 ± 0.20 | 1.44 ± 0.28 | 1.49 ± 0.25 | 1.50 ± 0.36 |
1.50 ± 0.27 | 1.47 ± 0.23 | 1.50 ± 0.30 | |||||
Adduction | 0.67 ± 0.38 | 0.61 ± 0.28 | 0.61 ± 0.34 | 0.52 ± 0.25 | 0.66 ± 0.38 | 0.64 ± 0.26 | |
0.64 ± 0.33 | 0.56 ± 0.29 | 0.65 ± 0.32* |
3.1. Knee Joint Load in the Sagittal Plane
There was no significant main effect of chair height (p = 0.48;
= 0.05) or limb dominance (p = 0.84;
< 0.01) on the peak external flexion moment. Likewise, there was no significant interaction effect of chair height and limb dominance (p = 0.41;
= 0.06; Fig. 2).
Chair height had a significant main effect on anterior knee shear force (p = 0.01;
= 0.27). However, there was no significant main effect of limb dominance (p = 0.43;
= 0.05) nor a significant interaction effect of chair height and limb dominance (p = 0.18;
= 0.12). Post-hoc analysis showed that low chair heights caused significantly higher anterior shear forces (p = 0.02).
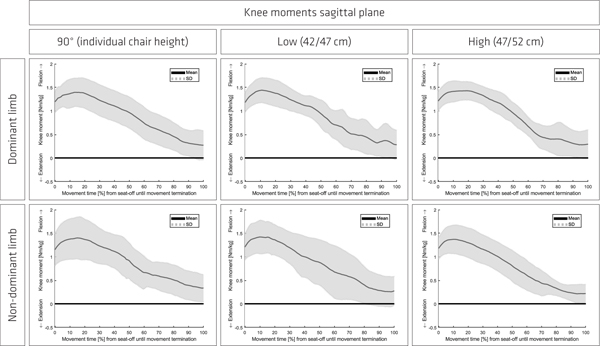
3.2. Knee Joint Load in the Frontal Plane
In terms of peak adduction moment, there was a significant effect of chair height (p = 0.02;
= 0.33) but not limb dominance (p = 0.44;
= 0.04). The interaction effect of chair height and limb dominance was not significant (p = 0.34;
= 0.07). Post-hoc analysis showed significantly lower peak adduction moments in the high chair height condition compared to the low chair height condition (p < 0.01, Fig. 3).
The peak lateral shear force at the knee was significantly affected by chair height (p < 0.01;
= 0.43) and the interaction of chair height and limb dominance (p = 0.04;
= 0.2), but not limb dominance itself (p = 0.32;
= 0.07). Post-hoc analysis showed significantly lower peak shear forces for high chair height than for low height (p < 0.01) and for high chair height than for the 90° condition (p = 0.02).
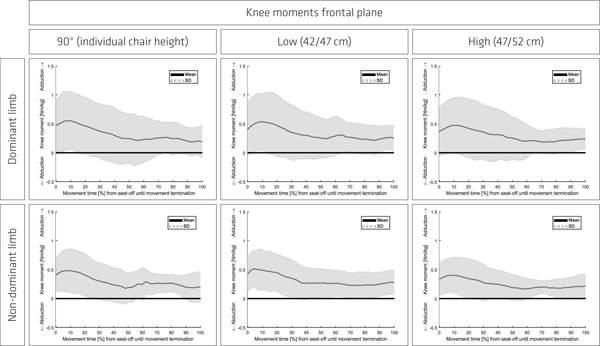
3.3. Level of Perceived Exertion
Individual exertion ratings ranged from 7.1 (extremely light) to 18.1 (very/extremely hard). The mean level of perceived exertion was 11.1 (± 2.8) for the high chair height, 12.5 (± 3.5) for the low chair height and 13.5 (± 3.1) in the 90° chair height condition. For the dominant and non-dominant lower limb, the mean ratings were 12.3 (± 3.3) and 12.3 (± 3.2) respectively, a non-significant difference (p = 0.19). The Friedman test revealed that chair height had a significant main effect on the level of perceived exertion (p < 0.01). Subsequent pairwise comparison showed that rising from the high chair height was perceived as less effort than rising from the low height (p = 0.03) and the 90° condition (p < 0.01).
4. DISCUSSION
To the best of our knowledge, this is the first study to investigate knee joint load during a unilateral STS task. The present study revealed the following main findings: (I) chair height had a significant impact on the peak adduction moment as well as on the anterior and lateral peak shear forces acting at the knee joint during the unilateral STS movement: Forces and moments were significantly lower at higher chair heights. (II) Lower limb dominance had no effect on knee joint load, determined via peak knee moments and forces. (III) Chair height but not limb dominance significantly affected the level of perceived exertion during the execution of unilateral STS movements.
4.1. Unilateral STS Movement as a Motor Skill Test in Health-related Sports or Sports for the Elderly
Various test systems are currently used to diagnose motor ability levels in health-related sports as well as sports for the elderly [5]. With regard to the increased inter-individual variability in motor abilities in middle [6, 18] and late [18, 19] adulthood, the scalability of motor test difficulty is an important aspect when creating test variants appropriate for the intended subject population.
The results of this study show that the unilateral STS movement was subjectively perceived as moderately strenuous with mean ratings between 11 and 13 on the Borg scale. This indicates the feasibility of this movement task for the population under investigation. However, the inter-individual differences in motor performance become apparent in the wide range of individual exertion ratings. These findings stress the need for scalable motor tests and the requirement of motor tests with difficulty levels beyond those of bilateral STS tests.
In terms of the ecological validity of the unilateral STS motor test in comparison to ADLs, it is acknowledged that most everyday STS movements are executed bilaterally. However, there are several everyday situations, e.g. car egress, which are performed unilaterally by all subjects in early adulthood and even most of the subjects in late adulthood [36]. As motor tests require rigorous objectivity, ADL movements like car egress [36] with its many degrees of freedom are not as suitable as the unilateral STS movement, which can be controlled more easily.
4.2. Knee Joint Load During the Unilateral STS Movement
Peak moments and forces are frequently used to quantify knee joint load [37, 38]. During the unilateral STS movement, particularly high external flexion (1.44-1.52 Nm/kg) and adduction moments (0.52-0.67 Nm/kg) as well as anterior (3.90-4.19 N/kg) and lateral (1.43-1.94 N/kg) shear forces were observed.
Other studies analysing the dynamics of ADLs reported peak flexion moments of 0.7-1.5 Nm/kg during stair ascent, 0.5 Nm/kg during level walking [39] and 0.4 Nm/kg during the bilateral STS movement with various knee angles [26, 40]. Thus, the peak flexion moments observed during the unilateral STS movement are comparable to those reported for stair ascent, which at times also requires unilateral lifting of the body weight. However, the peak knee flexion moments are three to four times higher than during level walking or the bilateral STS movement. The increased knee flexion moments in unilateral STS movements compared to level walking can rationally be attributed to the increased distance between the support area and the COM due to its more posterior position, causing a larger lever arm for the ground reaction force vector. Similarly, increased knee flexion moments in unilateral STS movements compared to bilateral STS movements are caused as the moment produced by the COM around the knee joint is not allocated to two but only one knee joint. The peak knee adduction moments during the unilateral STS movement are similar to those described for stair ascent [39]. The peak knee anterior shear forces of 4.7 N/kg during stair ascent and 3.2 N/kg during level walking [39] are slightly higher and lower, respectively, than those found in the present study. Finally, the peak lateral shear force is, in either case, higher than during stair ascent (1.3 N/kg) or level walking (1.5 N/kg) [39].
In summary, it can be stated that the forces and moments acting at the knee joint during the unilateral STS movement are higher than those previously described for level walking but similar to those for stair ascent. However, it should be considered that joint angles during stair ascent are not directly comparable to those during the unilateral STS movement [40] and an altered impact on the underlying biological structures is therefore presumed [41].
4.3. Effect of Chair Height on Knee Joint Load During the Unilateral STS Movement
Chair height influenced peak adduction moment as well as peak anterior and lateral shear forces acting at the knee joint. High chair height resulted in significantly lower peak adduction moments than the low chair height. We suspect this to be due to an increased lateral evasive movement of the knee during the unilateral STS movement from the low chair height leading to a more medial course of the ground reaction force vector. These findings might be important when executing STS movements with subjects suffering from medial compartment knee osteoarthrosis, as increased knee adduction moments have been associated with increased medial compartment knee joint load [42]. The increasing anterior shear forces observed with decreasing chair height might be explained by a greater contraction of the quadriceps muscle. This presumption is supported by findings of increasing vastus lateralis activity with decreasing chair height during the bilateral STS movement [20].
Altogether, these results are consistent with previous studies on the bilateral STS movement [20, 21, 27], which have reported increasing moments and task difficulty with reduced chair height.
4.4. Effect of Lower Limb Dominance on Knee Joint Load During the Unilateral STS Movement
Despite the asymmetries in knee extensor muscle strength reported in several studies of comparably-aged subject groups [32, 33] no differences were found between the dominant and non-dominant limb in this study. Therefore, the results suggest that lower limb dominance has no impact on knee joint load during the unilateral STS movement within the selected experimental conditions and population.
4.5. Limitations
The present study is the first to investigate knee joint load during the unilateral STS movements frequently included in motor test profiles [17, 43]. However, it has to be considered that in the majority of biomechanical studies, with exception of those using instrumented joint replacements [44], knee forces and moments are calculated using the inverse dynamics approach. Therefore, comparability of study results always depends on the applied signal processing routines (e.g. digital filtering) and the inverse dynamics model used. These issues most likely influence the calculated forces and moments. For this reason, the signal processing methods used in this study were geared towards the relevant literature [45] and the authors used a biomechanical model widespread in the field of clinical biomechanics [46]. However, there are several underlying simplifications to the Plug-in Gait model [35] used in the present study, as there are to any biomechanical model of the human body. The issues emerging from these simplifications are discussed in a number of publications along with the benefit of a widespread distribution of this model in clinical biomechanics. There is, therefore, a certain comparability of published study results [46]. Moreover, the present study focused on a population of healthy, middle-aged individuals with normal weight. The investigated population was generally able to perform a unilateral STS movement and the observed loads seemed acceptable from the authors’ point of view, especially as the unilateral STS movement is not intended to be performed in a high volume repetitive manner in the context of a motor test [17]. In addition, the average level of perceived exertion did not exceed 13.5 in any of the investigated conditions, representing medium perceived exertion.
CONCLUSION
The present study revealed the following main findings: (I) Forces and moments acting at the knee joint during a unilateral STS movement are similar to those provoked by other ADLs. (II) Forces and moments acting at the knee joint as well as the subjectively perceived exertion increase with decreasing chair height. (II) Lower limb dominance has no effect on the knee joint load.
These results document the objective and subjective feasibility of the unilateral STS movement as well as the tenability of the arising loads for the musculoskeletal system and therefore its suitability as a motor test in the population under investigation. However, these results cannot be generalized to e.g. obese or older (> 65 years) populations. For these populations, further investigations are needed to quantify knee joint loads during the bi- and unilateral STS movement from various chair heights.
ETHICS APPROVAL AND CONSENT TO PARTICIPATE
The study was approved by the Institutional Review Board.
HUMAN AND ANIMAL RIGHTS
No animals were used for this study. All humans research procedures performed in the current study were in accordance with the ethical standards of the institutional and/or national research committee and with the 1964 Helsinki declaration and its later amendments or comparable ethical standards.
CONSENT FOR PUBLICATION
All participants were informed about the protocol and gave their written informed consent before participating in the study.
CONFLICT OF INTEREST
The authors declare no conflict of interest, financial or otherwise.
ACKNOWLEDGEMENTS
We acknowledge support from Deutsche Forschungsgemeinschaft and Open Access Publishing Fund of Karlsruhe Institute of Technology. Additionally, we sincerely thank K. Franke and K. Wöhlken for their support during the data acquisition process.