All published articles of this journal are available on ScienceDirect.
Relationship Between Knee Valgus and Ground Reaction Force in Soccer Players Using Soccer Boots Landing on an Official Artificial Turf
Abstract
Introduction:
There is a high incidence of Anterior Cruciate Ligament (ACL) injuries in soccer and 37% of this happens during landing after a jumping event. The measure of valgus knee moment during landing of a Drop Jump (DJ) has been considered a gold standard test to predict the risk of ACL injury in young athletes. Furthermore, researchers have used 2D frontal angle of the knee trying to make a practical tool to evaluate this injury risk, however, to the best of our knowledge, there is no studies about the relationship between mechanical load and 2D dynamic knee valgus parameters.
Purpose:
To verify the relationship between kinetics and kinematics ACL injury risk factors: the GRF and the a) peak knee valgus; b) valgus knee displacement in soccer players wearing soccer boots landing on an official synthetic turf.
Methods:
Twenty Brazilian soccer players, 15-17 years old, with 176.6 ± 6.4 cm of height and 67.4 ± 8.1 kg of body mass participated in this study. Following familiarization, subjects performed the DJ from a height of 40 cm. They landed on two force plates synchronized with Vicon system for kinetic and kinematic analyses, respectively.
Results:
Only valgus knee displacement (-1.4 ± 7.0 °) and antero-posterior component of GRF (-0.402 ± 0.097 BW) presented a significant relationship (r = 0.353; p = 0.025).
Conclusion:
There is a significant correlation between knee valgus displacement and GRF antero-posterior component for soccer players in an official artificial turf using soccer boots.
1. INTRODUCTION
A high incidence of non-contact Anterior Cruciate Ligament (ACL) injuries in sports with cutting, jumping, sidestepping and pivoting movements is known [1, 2]. Non-contact ACL injuries are known to occur in the acceleration or deceleration phases of sports movement coupled with excessive quadriceps contraction, reduced hamstrings co-contraction and near knee full extension [3]. Furthermore, a higher ACL loading is due to a combination of internal/external rotation with dynamic knee valgus [3], a lower knee flexion and higher posterior ground reaction force [4]; and an increased knee abduction moment during landing impact [5]. The knee valgus has been described as a bodily position in which the knee collapses medially from excessive valgus or internal-external rotation or both [6].
The landing surface has been found to be another risk factor for ACL injuries. When compared to natural grass, artificial turf has higher chances to induce ACL injuries [7], mainly in male athletes [8]. Among these injuries, 37% happens during landing after a jumping event [9]. Furthermore, the player-surface interaction (i.e., the synthetic turf and the soccer boots) can increase joints mechanical overload as well as decrease in performance tests [10-12].
Biomechanics research about stress and injuries required to establish relationship between exercise and kinetic parameters [13]. The action of Ground Reaction Force (GRF) on body during landing, can be a decisive factor to occurrence of injuries [14]. The analysis of real situations where there were ACL ruptures in 3 soccer players demonstrated the angles and forces during injury: from -7 ° to 8 ° of valgus; from 33 ° to 73 ° of flexion; from -3 ° to 4 ° of internal rotation; maximum vertical GRF from 2.5 to 3.8 times body weight (BW); anterior-posterior component of the GRF from -2.6 to -0.5 times body weight; medial-lateral component of the GRF from -1.8 to 1.6 times body weight. This approach has the potential to generate a better understanding of injury mechanism although there are some limitations that have to be considered according to the authors [15]. Of interest is whether other variables that can be easily administered and calculated without a force plate, can be of diagnostic value. For this, the relationship between GRF parameters and field tests parameters should be investigated to assess a possible association and identify more suitable tests for practical applications.
The measure of valgus moment during landing of the Drop Jump (DJ) have been recommended [5] and considered a gold standard to predict ACL injuries. Indeed, Hewett et al. [16] showed a predictive effect (r2 = 0.88) to an increased risk of ACL injury in young athletes. Thus, the commonly used tool to establish an ACL risk of injury, the analysis of the dynamic valgus magnitude during landing of a DJ [16, 17], could be applied on a professional level synthetic grass in order to verify the risk of ACL injury and characterize the magnitudes of the mechanical overloads.
Researchers have used 2D frontal angle of the knee while landing trying to make a practical tool to evaluate [17-20]. These studies have used discreet angle values, either peak knee valgus [19, 20] or valgus knee displacement [17, 18], to assess ACL injury risk. However, to the best of our knowledge, there are no studies relating mechanical loading and 2D knee frontal angle parameters.
Therefore, the aim of this study was to verify the relationship between kinetics and kinematics ACL injury risk factors: the GRF and the a) peak knee valgus; b) valgus knee displacement in soccer players wearing soccer boots landing on an official synthetic turf.
2. METHODS
2.1. Subjects
Brazilian soccer players (n = 20; age = 16.3 ± 0.7 years old; height = 176.6 ± 6.4 cm; body mass: 67.4 ± 8.1 Kg) participated in this study. All participants used their own soccer boots to avoid the effect of non-familiarization with footwear [21, 22]. The participants were informed of the objectives and the methodological procedures as well as the possible risks and discomforts related to their participation in the study. Participants and parents or legal guardians signed assent and/or consent forms as appropriate. The local Ethics Committee approved all procedures.
2.2. Experimental Design
Initially, the subjects undertook a warm up and familiarization process with the Drop Jump (DJ) with a height of 40 cm. Six DJ's were performed on two boxes with an artificial turf recommended for stadiums elite clubs in international matches (“FIFA Quality Concept for Football Turf”). Above the artificial turf (2-Star® Sportlink International; São Paulo, Brazil) 14 kg/m2 of silica with 0.5-1.0 mm (Mineração Descalvado; Descalvado, Brazil) and 17 kg/m2 of rubber granules with 0.5-1.5 mm (Ecociclo; São Paulo, Brazil) were applied (Fig. 1). Then, each box with artificial turf was placed on top of two force plates (AMTI BP600900; Watertown, EUA). All DJ’s were recorded by five cameras (VICON MX3+; Oxford, UK) in order to obtain 3D joints’ analysis.
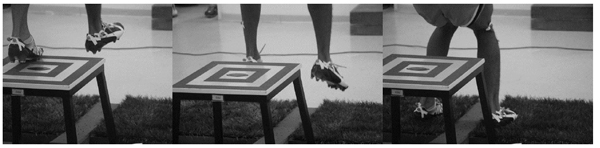
2.3. Testing Protocol
The DJ started with the subject on top of a 40 cm bench. The subjects were instructed to drop directly down off the bench and perform a maximum vertical jump as fast as they can. The subjects were required to land with one foot in each force plate. The two force plates recorded Ground Reaction Force (GRF) data at 2000 Hz and were synchronized with the Vicon system. Kinematic data was recorded at 200 Hz. Reflective markers were placed on the following anatomical points of the right and left hemispheres of the subjects: second metatarsal head, lateral malleolus, calcaneus, tibia, lateral femoral epicondyles, thigh, anterior superior iliac spine and sacrum. The Vicon Plugin Gait model was used to analyse the data.
2.4. Primary Outcome Measure
The peak value of each GRF component (i.e. antero-posterior component = Fx; mediolateral component = Fy; vertical component = Fz) and the peak knee valgus, as the higher adduction-abduction planar angle, of each trial was registered. Additionally, the valgus knee displacement was measured as the difference between the knee angle at landing moment and the peak knee angle during the contact phase. Six successful trials were recorded for each subject and the mean of all trials was used for further analysis [23, 24]. The DJ has high within-session reliability with intra-class correlation coefficients greater than 0.93 [25].
2.5. Statistical Analysis
Means and standard deviations were used as measures of centrality and spread of data. Normality was tested using the Kolmogorov-Smirnov tests. A Pearson correlation was calculated between knee abduction angle and GRF components. The intraclass correlation coefficient (ICC 1.k) data was calculated according to Weir [26]. A significance level of 0.05 was assumed (α=0.05) in all statistical tests. Statistical analysis of the data was performed on SigmaStat software 3.5.
3. RESULTS
The reliability data from select variables were: peak knee valgus (ICC = 0.996); knee valgus displacement (ICC = 0.997); antero-posterior component (ICC = 0.907); mediolateral component (ICC = 0.996); vertical component (ICC = 0.985). The GRF values of soccer players landing on an official synthetic turf using soccer boots were: -0.402 ± 0.097 Body Weight (BW) for antero-posterior component, 0.290 ± 0.094 BW for mediolateral component and 2.671 ± 1.156 BW for vertical component. Knee valgus dynamic parameters were: -1.4 ° ± 7.0 ° for valgus knee displacement and 7.1 ° ± 11.7 ° for peak knee valgus.
Relationship between GRF and knee valgus dynamic parameters is shown in Fig. (2).
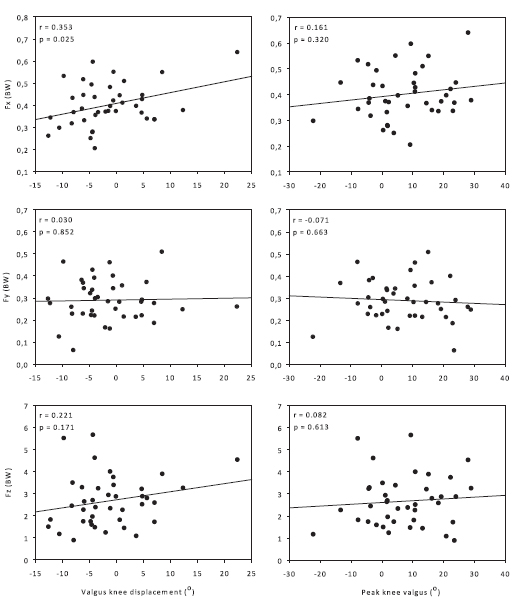
4. DISCUSSION
The aim of this study was to verify the relationship between GRF peak and knee valgus dynamic parameters in soccer players using an official synthetic turf and soccer boots. Only valgus knee displacement and antero-posterior component of GRF presented a significant relationship (p = 0.025).
The factors associated with high mechanical ACL overload are still controversial. A combination of both internal and external rotation with dynamic valgus increases the injury risk, wherein the internal rotation has showed higher odds [3]. The increased knee abduction moment (valgus intersegmental torque) during landing is also associated with an increased injury risk [5]. However, YU & GARRETT [4] found no evidence that knee valgus-varus and internal-external rotation moments can produce non-contact ACL injuries. In fact, decreased knee flexion angle with increased quadriceps muscle force and posterior ground reaction force, causing an increased knee extension moment, are requirements for increased ACL loading [4].
The present study found a significant relationship between valgus knee displacement and GRF antero-posterior component. When HEWETT et al. [16] used 3D video analysis, significant correlations between knee adduction angle and peak vertical GRF were observed in ACL injured (r = 0.67, p < 0.001), but not for uninjured athletes (p = 0.44). In field tests using 2D video analysis, either peak angle [19, 20] or valgus knee displacement [17, 18] are used to analyse ACL injury risk. Additionally, a great posterior ground reaction force is also related with increased ACL injury risk [4]. Based on these findings as well as on our results, valgus knee displacement should be recommended for field tests, since it has a significant relationship with GRF antero-posterior component.
This increased risk has also been verified when the game is played on artificial turf [7]. Furthermore, the player-surface interaction on artificial soccer turf seems to have an influence on mechanical overload in joints and in testing performance [10-12]. In order to bring more specific conditions for the testing protocol, in the present study the subjects performed DJ on surface of artificial turf recommended for “FIFA Quality Concept for Football Turf”. To the best of our knowledge, studies with similar approach were not found. BUTLER, RUSSELL & QUEEN [10] found 1.94 ± 0.33 BW for male and 1.72 ± 0.37 BW for female soccer players during a jump test to hit a soccer ball with the head at 50% of their maximum vertical jump height on artificial turf with soccer boots. However, they did not describe if the FIFA recommendation to artificial turf was attended and the DJ was not performed. On the other hand, HEWETT et al. [16] performed the DJ dropping off from a 31 cm box with injured and non-injured ACL athletes and found GRF vertical component of 2.098 ± 1.841 BW and 1.824 ± 3.648 BW, respectively. However, the DJ was performed on a rigid surface (i.e. force plate without artificial turf). These different setups did not allow comparing our results with current literature. There are some limitations in the present study: it was not compared artificial turf and rigid surface effects on mechanical overload or as soccer boots with conventional shoes.
CONCLUSION
The relationship between knee valgus displacement and GRF antero-posterior component for soccer players landing on an official artificial turf using soccer boots was significant. Practical Application: in DJ test with just 2D video analysis, knee valgus displacement is better than peak knee valgus to indicate ACL injury risk.
ETHICS APPROVAL AND CONSENT TO PARTICIPATE
The local Ethics Committee approved all procedures. (CAAE: 20507413.9.0000.5391) (Approved: 08/04/2014)
HUMAN AND ANIMAL RIGHTS
No animals were used for this study. All humans research procedures performed in the current study were in accordance with the ethical standards of the institutional and/or national research committee and with the 1964 Helsinki declaration and its later amendments or comparable ethical standards.
CONSENT FOR PUBLICATION
The participants were informed of the objectives and the methodological procedures as well as the possible risks and discomforts related to their participation in the study. Participants and parents or legal guardians signed assent and/or consent forms as appropriate.
CONFLICTS OF INTEREST
The authors declare no conflict of interest, financial or otherwise.
ACKNOWLEDGEMENTS
Declared none.